Food a delicious science offers an exciting exploration of how culinary arts intersect with scientific principles. This journey begins by examining the fundamental chemistry and physics that govern cooking, from heat transfer’s influence on different cooking methods to the transformative effects of molecular gastronomy. The essence of flavor, texture, preservation, and cooking techniques will be explored, revealing the intricate science behind every delicious bite.
We will delve into the building blocks of flavor, dissecting the chemical compositions of ingredients like proteins, carbohydrates, and fats. You will learn about the Maillard reaction and how volatile compounds shape aromas and influence taste. Furthermore, the art of creating textures will be uncovered, examining how proteins impact the mouthfeel of foods, and how to achieve perfect crispy exteriors and tender interiors in recipes.
Finally, we’ll explore the science behind food preservation and the exciting future of food innovation and technology.
The Culinary Arts as a Scientific Discipline
The culinary arts, at their core, are a practical application of scientific principles. Cooking is not merely a creative endeavor but a controlled experiment where ingredients interact under specific conditions to produce desired outcomes. Understanding the underlying science is crucial for chefs and home cooks alike to consistently achieve predictable and delicious results. This understanding allows for the optimization of cooking processes, leading to enhanced flavors, textures, and overall culinary experiences.
Chemistry and Physics in Cooking
Cooking relies heavily on the principles of chemistry and physics. From the simplest tasks to the most complex dishes, these sciences dictate how ingredients transform and interact. Chemical reactions, such as oxidation and reduction, and physical processes, like heat transfer and phase changes, are fundamental to the art of cooking.
- Chemical Reactions: The Maillard reaction, a non-enzymatic browning reaction, is a prime example of chemistry at work. This reaction occurs between amino acids and reducing sugars at high temperatures, resulting in the browning of food and the development of complex flavors. This is why a perfectly seared steak tastes so good.
- Protein Denaturation: Heating proteins causes them to denature, unfolding and changing their structure. This process is essential for cooking eggs, meat, and other protein-rich foods. For example, when an egg is cooked, the proteins coagulate, creating a solid texture.
- Caramelization: The browning of sugars when heated, caramelization, is another key chemical reaction. This process produces rich, complex flavors and colors. Caramelization is used to make caramel sauce, candies, and other sweet treats.
- Emulsification: Emulsions, such as mayonnaise and vinaigrette, are mixtures of two immiscible liquids, like oil and water. Emulsifiers, such as egg yolks in mayonnaise, stabilize the mixture, preventing the liquids from separating.
Heat Transfer and Cooking Methods
Heat transfer is a critical aspect of cooking, and different cooking methods utilize different modes of heat transfer. Understanding these modes allows for precise control over cooking processes and desired outcomes. The three primary modes of heat transfer are conduction, convection, and radiation.
- Conduction: This is the transfer of heat through direct contact. Cooking on a stovetop, using a pan, is an example of conduction. The heat from the burner transfers to the pan, and then to the food.
- Convection: Convection involves the transfer of heat through the movement of fluids (liquids or gases). Baking in an oven utilizes convection; hot air circulates around the food, cooking it evenly. Boiling and deep-frying also rely on convection.
- Radiation: Radiation is the transfer of heat through electromagnetic waves. Broiling and grilling utilize radiation, where heat is transferred directly from a heat source to the food.
- Different Cooking Methods: Each cooking method utilizes a combination of these heat transfer modes.
- Baking: Primarily uses convection, with some conduction from the baking pan.
- Grilling: Primarily uses radiation, with some conduction from the grill grates.
- Frying: Primarily uses convection, as the food is surrounded by hot oil.
Molecular Gastronomy Techniques
Molecular gastronomy employs scientific principles to transform food textures and flavors in innovative ways. This approach uses techniques derived from physics and chemistry to create unique culinary experiences. Some of these techniques are now commonplace in modern kitchens.
- Spherification: This technique involves encapsulating a liquid in a spherical shape, creating “caviar” or “pearls” of flavor. This is achieved by adding a sodium alginate solution to a liquid and dropping it into a calcium chloride bath, causing the alginate to form a gel around the liquid.
- Reverse Spherification: This is the opposite of spherification, where a calcium chloride solution is added to the food, and then dropped into a sodium alginate bath.
- Emulsification: As mentioned previously, this is also a molecular gastronomy technique, especially in creating foams and airs. By using specific emulsifiers and techniques, chefs can create light, airy textures.
- Sous Vide: This technique involves cooking food in a precisely controlled water bath at a low temperature for an extended period. This results in even cooking and enhanced flavor retention.
- Foams and Airs: These are created using emulsifiers and often a siphon. They add lightness and a burst of flavor to a dish.
Ingredients
Ingredients are the fundamental components of any culinary creation, acting as the building blocks that determine a dish’s flavor, texture, and overall appeal. Understanding their chemical composition and how they interact during cooking is crucial for achieving desired results and pushing the boundaries of culinary innovation.
Chemical Composition of Common Ingredients
The flavor, texture, and nutritional value of ingredients are profoundly influenced by their chemical makeup. Proteins, carbohydrates, and fats are the major macronutrients that dictate these characteristics.
Proteins are complex molecules composed of amino acids linked by peptide bonds. They are essential for building and repairing tissues and play a critical role in enzyme function. Consider the differences in texture and structure between:
- Meat: Primarily composed of proteins like actin and myosin, which contribute to its fibrous texture. Heat denatures these proteins, causing them to coagulate and firm up, which is the basis of cooking meat.
- Eggs: The proteins in egg whites, mainly ovalbumin, coagulate upon heating, creating a solid structure. This process is crucial for baking and thickening sauces.
Carbohydrates are composed of sugars, starches, and fibers. They provide energy and contribute to sweetness, texture, and browning in food. Different forms of carbohydrates have distinct impacts:
- Sugars (glucose, fructose, sucrose): Contribute sweetness and can undergo caramelization upon heating, which results in complex flavor compounds.
- Starches (amylose, amylopectin): Found in grains and vegetables. Starches gelatinize when heated in the presence of water, which thickens sauces and creates the structure in baked goods.
- Fiber (cellulose, hemicellulose): Provides structure in plant-based foods and is indigestible by humans. It affects texture and can influence how other ingredients interact.
Fats (lipids) are composed of triglycerides, which are molecules of glycerol bonded to three fatty acids. They contribute flavor, texture, and energy. The type of fatty acid determines the fat’s properties:
- Saturated fats: Tend to be solid at room temperature and are often associated with richer flavors.
- Unsaturated fats: Liquid at room temperature. They contribute to tenderness and can be healthier choices.
Impact of Cooking Fats on Flavor and Texture
Different cooking fats have varying impacts on flavor and texture due to their unique compositions and behavior at different temperatures. The choice of fat significantly affects the final characteristics of a dish.
The flavor profiles of different fats are quite diverse, influencing the overall taste of the food:
- Butter: Adds a rich, creamy flavor and contributes to a tender crumb in baked goods. Its milk solids can also brown and add complexity to flavor.
- Olive Oil: Offers a fruity or peppery flavor depending on the variety and can add moisture and a subtle taste to dishes.
- Coconut Oil: Imparts a distinct coconut flavor, which can be desirable in some dishes, and contributes to a unique texture.
- Vegetable Oils (canola, sunflower, etc.): Are relatively neutral in flavor, allowing the other ingredients to be the stars of the show. They can also withstand higher temperatures, making them ideal for frying.
The texture is also affected by the fat used in cooking. Consider the differences:
- Shortening: Often used in baking because of its high saturated fat content. It creates a flaky texture in pie crusts and biscuits.
- Lard: Another animal fat, also high in saturated fat. It produces a tender and flavorful crust.
- Frying Oils: The type of oil used affects the crispness and moisture of fried foods. Oils with higher smoke points are suitable for frying.
Role of Enzymes in the Ripening of Fruits and Vegetables
Enzymes, biological catalysts, play a crucial role in the ripening of fruits and vegetables. They initiate and accelerate chemical reactions that transform the flavor, texture, and appearance of produce.
Enzymes contribute to the changes during ripening:
- Pectinases: Break down pectin, a structural polysaccharide found in cell walls, leading to softening of the fruit.
- Amylases: Convert starches into sugars, increasing the sweetness of the fruit.
- Ethylene: A gaseous hormone that acts as a ripening agent, triggering the production of other enzymes.
These enzymatic reactions lead to noticeable changes:
- Flavor Development: The breakdown of starches into sugars (like glucose and fructose) by amylases increases the sweetness of the fruit. Acids are also broken down, decreasing tartness.
- Texture Modification: Pectinases break down pectin, causing the fruit to soften.
- Color Changes: Chlorophyll breaks down, revealing other pigments, like carotenoids (yellow/orange) and anthocyanins (red/purple). For example, the green skin of a green banana becomes yellow as chlorophyll degrades.
Flavor: A Symphony of the Senses
Flavor is a complex sensory experience, far exceeding the simple combination of taste and smell. It is a holistic perception, integrating taste, aroma, texture, temperature, and even visual cues to create a complete gustatory profile. Understanding the intricate interplay of these elements is fundamental to the culinary arts and the science of food.
The Maillard Reaction and Flavor Development
The Maillard reaction is a complex chemical process that occurs between amino acids and reducing sugars when heated, typically above 140°C (284°F). This non-enzymatic browning reaction is responsible for the characteristic flavors and aromas of many cooked foods, from the crust of bread to the sear on a steak.
- The Maillard reaction involves a series of intricate steps, beginning with the initial reaction between a reducing sugar (such as glucose or fructose) and an amino acid.
- This initial reaction produces unstable intermediates, which then undergo further transformations, leading to the formation of a diverse range of flavor compounds. These compounds include aldehydes, ketones, furans, pyrazines, and many others, each contributing to the overall flavor profile.
- The specific flavor compounds produced depend on factors such as temperature, pH, the type of amino acids and sugars present, and the duration of heating. For instance, high-heat cooking, like searing, promotes the formation of different flavor compounds than slow-cooking methods.
- The Maillard reaction is not always desirable; excessive browning can lead to the formation of undesirable bitter or burnt flavors.
- Examples of the Maillard reaction’s impact are evident in the browning of roasted coffee beans, the crust of baked bread, and the surface of grilled meats. The complex flavors that develop are a direct result of this reaction.
Volatile Compounds, Aromas, and Taste Perception
Aromas, primarily conveyed by volatile compounds, play a crucial role in flavor perception. These airborne molecules stimulate the olfactory receptors in the nasal cavity, contributing significantly to the overall sensory experience of food. The interplay between aroma and taste creates a unified perception of flavor.
- Volatile compounds are chemical substances with a low boiling point, allowing them to evaporate easily and reach the olfactory receptors.
- The aroma of a food is a complex mixture of these volatile compounds, each contributing a unique scent note.
- These compounds are detected by olfactory receptors, which send signals to the brain, where the aroma is interpreted.
- Aromas can significantly influence taste perception. For instance, the same food can taste vastly different when the nose is blocked, as the aroma component is diminished.
- Examples include the floral aroma of jasmine rice, the fruity aroma of ripe mangoes, and the savory aroma of freshly brewed coffee. These aromas, in combination with taste, contribute significantly to the overall flavor experience.
Experiment: Impact of Salt on Perceived Sweetness
Salt can enhance the perception of sweetness in foods. This experiment aims to investigate the effect of varying salt concentrations on the perceived sweetness of a simple solution.
- Objective: To determine how different concentrations of salt affect the perceived sweetness of a sucrose solution.
- Materials:
- Sucrose (table sugar)
- Table salt (sodium chloride)
- Distilled water
- Beakers or small cups
- Measuring spoons or graduated cylinders
- Tasters (participants)
- Procedure:
- Prepare a base sucrose solution of a known concentration (e.g., 5% sucrose) in distilled water.
- Divide the base sucrose solution into several beakers.
- Add different concentrations of salt to each beaker. Examples include: 0% salt (control), 0.1% salt, 0.2% salt, and 0.3% salt. Ensure thorough mixing.
- Have the tasters sample each solution, starting with the control (no salt).
- Ask the tasters to rate the perceived sweetness of each solution on a scale (e.g., 1-5, where 1 is “not sweet” and 5 is “very sweet”).
- Record the sweetness ratings for each salt concentration.
- Expected Results: The results are expected to show that as the salt concentration increases, the perceived sweetness of the sucrose solution also increases, up to a certain point. Higher salt concentrations might begin to mask the sweetness or introduce a salty taste that detracts from the sweetness. The data will likely reveal a correlation between salt concentration and perceived sweetness.
- Data Analysis: The average sweetness ratings for each salt concentration can be calculated. These averages can then be plotted on a graph, with salt concentration on the x-axis and perceived sweetness on the y-axis. This graph will visually represent the relationship between salt and perceived sweetness.
Texture: The Mouthfeel Factor: Food A Delicious Science
Texture significantly influences our perception of food, playing a crucial role in the overall dining experience. It’s the physical sensation that food elicits in our mouths, encompassing a wide range of properties from smoothness to crunchiness. Understanding and manipulating texture is fundamental in culinary arts, allowing chefs to create diverse and appealing dishes.Texture results from the interaction of food’s physical structure with our oral cavity.
Factors such as the arrangement of molecules, the presence of air pockets, and the cooking method employed all contribute to the final texture.
Textural Properties of Different Cooking Methods
Various cooking methods impart distinct textural characteristics to food. Each method affects the food’s internal structure, moisture content, and surface properties, leading to different mouthfeel sensations.
Get the entire information you require about health food stores cincinnati on this page.
Cooking Method | Typical Textural Properties | Examples | Scientific Basis |
---|---|---|---|
Boiling | Tender, sometimes soft or mushy, depending on the cooking time and the food’s composition. | Boiled potatoes, pasta, eggs. | High heat in water denatures proteins and softens cell walls, leading to a breakdown of structure. |
Frying | Crispy exterior, often with a tender interior. Can range from light and airy to dense and chewy, depending on the food and frying process. | Fried chicken, french fries, donuts. | Rapid surface dehydration creates a crust. Internal moisture steams the food, maintaining tenderness. Maillard reaction contributes to browning and flavor. |
Baking | Varies greatly depending on the recipe. Can be fluffy, chewy, crispy, or crumbly. | Bread, cakes, cookies. | Heat causes expansion of gases (CO2), protein coagulation, and starch gelatinization, impacting structure and texture. |
Grilling | Charred exterior with a slightly smoky flavor; can be tender or firm depending on the food and doneness. | Grilled steak, vegetables, chicken. | High heat from direct flame causes surface caramelization and protein denaturation, creating a seared crust. |
Achieving Crispy Exterior and Tender Interior in Fried Chicken
Creating fried chicken with a perfect balance of crispy skin and juicy meat involves several critical steps. These steps focus on controlling moisture, temperature, and the development of a flavorful crust.
- Brining the Chicken: Brining the chicken (soaking it in a saltwater solution) helps to season the meat throughout and retain moisture during frying. The salt denatures muscle proteins, allowing them to hold onto more water.
- Drying the Chicken: Thoroughly drying the chicken pieces after brining and before coating is crucial. Excess moisture prevents the crust from forming properly and can lead to a soggy exterior.
- Coating with Flour and Seasonings: A well-seasoned flour mixture creates the crispy crust. The flour provides the structure, while seasonings add flavor.
- Double-Dredging (Optional): Double-dredging (coating the chicken in flour, dipping it in a liquid like buttermilk or egg wash, and then coating it again in flour) can create a thicker, crispier crust. The liquid helps the flour adhere and creates a textured surface.
- Maintaining the Oil Temperature: Maintaining the oil temperature consistently at around 325-350°F (160-175°C) is critical. Too low, and the chicken will absorb too much oil, resulting in a greasy texture. Too high, and the exterior will burn before the interior is cooked through.
- Frying in Batches: Frying in batches prevents overcrowding the pot, which lowers the oil temperature. This allows the chicken to cook evenly.
- Resting After Frying: Allowing the fried chicken to rest on a wire rack after frying allows excess oil to drain and the crust to crisp further.
Protein Structure’s Effect on Egg Texture
The structure of proteins plays a significant role in determining the texture of cooked eggs. When eggs are heated, the proteins undergo a process called denaturation and coagulation, which changes their physical properties.
- Denaturation: When exposed to heat, egg proteins (primarily ovalbumin, found in the egg white) begin to unfold or denature. This disrupts the bonds that hold the protein molecules in their original shape.
- Coagulation: As the proteins denature, they begin to form new bonds with each other, creating a network or matrix. This process is known as coagulation. The extent of coagulation determines the firmness of the egg.
- Factors Influencing Coagulation: Several factors affect coagulation, including temperature and the presence of other ingredients. The temperature at which egg proteins coagulate varies depending on the specific protein and its environment. For example, egg whites coagulate at lower temperatures than egg yolks. The presence of acids, such as vinegar or lemon juice, can also affect coagulation, causing the proteins to set more quickly.
- Achieving Desired Textures: Controlling the cooking temperature is key to achieving the desired egg texture. For example:
- Soft-boiled eggs: Cooking at a gentle simmer allows the whites to set while the yolks remain soft.
- Hard-boiled eggs: Cooking at a higher temperature ensures that both the whites and yolks are fully coagulated.
- Scrambled eggs: Gentle heat and constant stirring create a creamy, tender texture by preventing the proteins from forming large, firm clumps. Adding a small amount of liquid, such as milk or cream, further softens the texture.
Preservation: Extending the Life of Food
Food preservation is a critical aspect of food science, allowing us to extend the shelf life of perishable items, reduce waste, and ensure a consistent food supply. It involves a range of techniques designed to inhibit microbial growth, slow down enzymatic reactions, and prevent spoilage caused by various factors. Understanding the science behind these methods is essential for both food producers and consumers.
Canning: High-Heat Sterilization
Canning is a preservation method that involves sealing food in airtight containers and then heating them to a temperature that destroys spoilage microorganisms and inactivates enzymes. This process creates a commercially sterile product, meaning it is free from microorganisms capable of growing in the food under normal storage conditions.The canning process typically includes the following steps:
- Preparation: Food is prepared (e.g., washing, peeling, chopping) and packed into clean jars or cans.
- Exhausting: Air is removed from the headspace of the container, either by preheating the food or by using a vacuum sealer. This helps to reduce pressure on the container during processing and minimize oxidation.
- Processing: The sealed containers are heated to a specific temperature for a specific time, depending on the food’s acidity and the size of the container. High-acid foods (e.g., fruits) can be processed at lower temperatures (e.g., boiling water), while low-acid foods (e.g., vegetables, meats) require higher temperatures (e.g., pressure canning) to kill
-Clostridium botulinum* spores, which can produce a deadly toxin. - Cooling: After processing, the containers are cooled rapidly to prevent overcooking and maintain product quality.
The effectiveness of canning depends on several factors, including the initial microbial load of the food, the pH of the food, the processing temperature and time, and the integrity of the container seal. For example, the higher the acidity of the food, the easier it is to preserve, as most spoilage bacteria cannot survive in acidic environments.
Pickling: Acidic Environment
Pickling involves preserving food in an acidic solution, typically vinegar, which inhibits the growth of spoilage microorganisms. The high acidity (low pH) of the pickling brine creates an environment that is unfavorable for most bacteria, yeasts, and molds.The pickling process usually involves:
- Preparation: Food is washed, trimmed, and sometimes pre-treated (e.g., blanching) to improve texture and reduce microbial load.
- Brining: The food is submerged in a brine solution, which typically contains vinegar, salt, and spices. The vinegar provides the acidity, the salt helps to draw moisture out of the food, and the spices add flavor.
- Fermentation (optional): Some pickles undergo a fermentation process, where beneficial bacteria (e.g.,
-Lactobacillus*) convert sugars into lactic acid, further increasing the acidity and contributing to the unique flavor of fermented pickles. - Sealing and Storage: Pickles are stored in airtight containers to prevent spoilage.
The pH of the pickling brine is a critical factor in determining the safety and shelf life of pickled foods. A pH of 4.6 or lower is generally considered safe for pickling, as it inhibits the growth ofC. botulinum*.
Fermentation: Controlled Microbial Activity
Fermentation is a preservation method that relies on the controlled growth of beneficial microorganisms to convert carbohydrates into acids, alcohols, or gases. This process not only preserves the food but also often enhances its flavor and nutritional value.Different types of fermentation processes include:
- Lactic Acid Fermentation: This is the most common type of fermentation used in food preservation. Lactic acid bacteria (LAB), such as
-Lactobacillus*, convert sugars into lactic acid, which lowers the pH and inhibits the growth of spoilage microorganisms. Examples include sauerkraut, kimchi, and yogurt. - Alcoholic Fermentation: Yeasts, such as
-Saccharomyces cerevisiae*, convert sugars into alcohol and carbon dioxide. This process is used to make alcoholic beverages like beer and wine. - Acetic Acid Fermentation: Acetic acid bacteria convert alcohol into acetic acid (vinegar).
The fermentation process is carefully controlled to ensure the growth of desirable microorganisms and to prevent the growth of spoilage organisms. Factors such as temperature, salt concentration, and the presence of oxygen can influence the type of fermentation that occurs. For example, in sauerkraut production, salt is added to inhibit the growth of undesirable bacteria and to promote the growth of LAB.
Impact on Nutritional Content, Food a delicious science
Different preservation methods can have varying effects on the nutritional content of food.Here are some examples:
- Canning: Heat processing during canning can lead to the loss of some water-soluble vitamins (e.g., vitamin C and B vitamins). However, the overall nutritional value of canned foods is often comparable to fresh or frozen foods. Some nutrients, like lycopene in tomatoes, become more bioavailable after canning.
- Pickling: Pickling can result in some loss of water-soluble vitamins, but it also adds beneficial bacteria in fermented pickles. The addition of salt can increase the sodium content of the food.
- Fermentation: Fermentation can enhance the nutritional value of food. For example, fermentation can increase the bioavailability of certain nutrients, such as iron and zinc. Fermented foods are also a good source of probiotics, which are beneficial bacteria that can promote gut health. Fermentation also produces B vitamins.
The specific impact of a preservation method on nutritional content depends on the food being preserved, the preservation technique used, and the processing conditions.
Microorganisms and Food Spoilage
Microorganisms play a crucial role in both food spoilage and food preservation. Food spoilage is primarily caused by the growth of microorganisms, including bacteria, yeasts, and molds, which produce enzymes that break down food components, leading to changes in taste, texture, and appearance.Common spoilage microorganisms include:
- Bacteria:
-Pseudomonas*,
-Bacillus*,
-Clostridium*, and
-Listeria* are examples of bacteria that can cause food spoilage. Some bacteria, such as
-Salmonella* and
-E. coli*, can also cause foodborne illness. - Yeasts: Yeasts can cause spoilage in foods with high sugar content, such as fruits and fruit juices.
- Molds: Molds can grow on a wide variety of foods and can produce mycotoxins, which are harmful substances.
Food preservation techniques aim to control the growth of these spoilage microorganisms. Canning, pickling, and fermentation all work by creating environments that are unfavorable for the growth of spoilage organisms.
Cooking Techniques: Precision and Control
The art of cooking, while often perceived as intuitive, is fundamentally a science. Mastering cooking techniques involves understanding and manipulating various factors to achieve desired outcomes in food preparation. This includes controlling heat, time, and the interactions between ingredients. Precise control over these elements is crucial for transforming raw ingredients into delicious and safe meals.
Sous Vide Cooking: Precise Temperature Control
Sous vide, meaning “under vacuum” in French, is a cooking technique that involves immersing food in a water bath at a precisely controlled temperature. This method allows for incredibly even cooking, as the entire surface of the food is exposed to the same temperature.The science behind sous vide relies on several key principles:
- Heat Transfer: Heat is transferred to the food through the water, which acts as a uniform heat conductor. Unlike conventional cooking methods, there are no hot spots or significant temperature gradients.
- Protein Denaturation: Proteins in food denature (unfold and change shape) at specific temperatures. Sous vide allows cooks to precisely control the degree of denaturation, resulting in desired textures. For example, meat cooked at a lower temperature for a longer time remains tender and juicy because less moisture is lost.
- Moisture Retention: Because the food is cooked in a vacuum-sealed bag, moisture loss is minimized. This helps to maintain the food’s natural flavors and textures.
- Pasteurization: Sous vide cooking can pasteurize food, killing harmful bacteria. The time and temperature required for pasteurization depend on the type of food and the desired level of safety. For example, the USDA recommends cooking poultry to an internal temperature of 165°F (74°C) to ensure food safety.
Heat Sources in Cooking: Comparative Analysis
Different heat sources offer varying levels of control, heat distribution, and efficiency. The choice of heat source significantly impacts the final product.
- Conduction: This involves the direct transfer of heat from a heat source to a cooking vessel and then to the food.
- Examples: Stovetops (gas, electric, induction), griddles, and skillets.
- Advantages: Relatively efficient, provides direct heat, good for searing and browning.
- Disadvantages: Can create hot spots, uneven cooking if not managed carefully.
- Convection: This involves the circulation of heated air around the food.
- Examples: Ovens (conventional and convection), air fryers.
- Advantages: More even cooking than conduction alone, can cook food faster.
- Disadvantages: Can dry out food if not managed properly.
- Radiation: This involves the transfer of heat through electromagnetic waves.
- Examples: Broilers, grills, microwaves.
- Advantages: Can cook food quickly, good for browning.
- Disadvantages: Can cook food unevenly, can dry out food quickly.
Each heat source has its own characteristics, and the best choice depends on the desired outcome and the type of food being cooked. Induction cooktops, for example, offer precise temperature control and rapid heating, making them ideal for delicate cooking tasks.
Optimal Cooking Temperatures for Meat: Achieving Desired Doneness
The internal temperature of meat is a crucial factor in determining its doneness and texture. Different cuts of meat and individual preferences necessitate specific cooking temperatures to achieve the desired result.The following table provides a guide to internal temperatures for various cuts of meat:
Meat Type | Doneness | Internal Temperature (°F) | Description |
---|---|---|---|
Beef | Rare | 125-130 | Red center, soft texture. |
Beef | Medium Rare | 130-140 | Warm red center, slightly firmer. |
Beef | Medium | 140-150 | Pink center, firmer texture. |
Beef | Medium Well | 150-160 | Slightly pink, firm texture. |
Beef | Well Done | 160+ | No pink, very firm texture. |
Pork | Medium | 145 | Slightly pink. |
Pork | Well Done | 160+ | No pink. |
Poultry (Chicken & Turkey) | Well Done | 165 | No pink, cooked throughout. |
Fish | Cooked | 145 | Opaque, flakes easily with a fork. |
It’s essential to use a reliable meat thermometer to accurately measure the internal temperature and ensure the meat is cooked to the desired doneness and safe to eat. For example, the USDA recommends cooking ground beef to an internal temperature of 160°F (71°C) to kill harmful bacteria.
Food and Health: Nutritional Science
Understanding the intricate relationship between food and health is crucial for making informed dietary choices. Nutritional science delves into the components of food, their impact on the body, and how they contribute to overall well-being. This section explores the influence of cooking on nutritional value, the essential roles of vitamins and minerals, and healthy cooking methods.
Impact of Cooking on Nutritional Value
Cooking significantly alters the nutritional content of food, with both positive and negative effects. The changes depend on the cooking method, temperature, and duration. Some nutrients are enhanced, while others are diminished. For instance, cooking can improve the bioavailability of certain nutrients, making them easier for the body to absorb. However, excessive heat can destroy heat-sensitive vitamins like vitamin C and some B vitamins.
Role of Vitamins and Minerals in Human Health
Vitamins and minerals are micronutrients essential for numerous bodily functions. They are vital for growth, development, and maintaining overall health. Vitamins are organic compounds, while minerals are inorganic elements.* Vitamins: These are crucial for various metabolic processes.
Vitamin A
Supports vision, immune function, and cell growth. Deficiencies can lead to night blindness and impaired immunity.
Vitamin C
Acts as an antioxidant, aids in wound healing, and boosts the immune system. Lack of Vitamin C leads to scurvy.
Vitamin D
Essential for calcium absorption and bone health. Insufficient levels can cause rickets in children and osteoporosis in adults.
Vitamin E
Protects cells from damage.
B Vitamins (B1, B2, B3, B6, B9, B12)
Play roles in energy production, nerve function, and cell metabolism. Deficiencies can lead to various health problems, including anemia and neurological issues.
Minerals
These are vital for structural and regulatory functions.
Calcium
Important for bone and teeth health, muscle function, and nerve transmission. Deficiency can lead to osteoporosis.
Iron
Carries oxygen in the blood. Iron deficiency causes anemia, resulting in fatigue and weakness.
Potassium
Helps regulate blood pressure and muscle function.
Zinc
Supports immune function, wound healing, and cell growth.
Healthy Cooking Methods that Preserve Nutrients
Employing specific cooking methods can minimize nutrient loss and maximize the nutritional value of food.
- Steaming: This method uses steam to cook food, preserving water-soluble vitamins like vitamin C and B vitamins. Vegetables retain their color, texture, and nutritional value.
- Stir-frying: Quick cooking at high heat with minimal oil helps retain nutrients. The brief cooking time minimizes nutrient degradation.
- Microwaving: This method can preserve nutrients because of the shorter cooking times and minimal water usage.
- Braising: A combination of dry and moist heat, braising is suitable for tougher cuts of meat, allowing them to become tender while retaining nutrients.
- Boiling/Poaching (with limitations): While some nutrients can leach into the water, these methods are still useful. The cooking liquid can be consumed (e.g., soups) to retain the nutrients.
- Roasting: Roasting can preserve nutrients. The browning of food creates flavorful compounds and reduces nutrient loss compared to high-temperature frying.
The Future of Food
The food industry is undergoing a significant transformation, driven by advancements in technology and a growing awareness of sustainability. These innovations are reshaping how we produce, process, and consume food, promising to address challenges like food security, environmental impact, and nutritional value. This exploration delves into the cutting-edge technologies and scientific advancements poised to revolutionize the culinary landscape.
Food Technology and Sustainable Food Systems
Food technology plays a crucial role in creating sustainable food systems. By leveraging scientific principles and technological innovations, we can optimize resource utilization, minimize waste, and reduce the environmental footprint of food production. This encompasses a wide range of applications, from precision agriculture to alternative protein sources.
- Precision Agriculture: This involves using data analytics, sensors, and automation to optimize farming practices. For example, drones equipped with multispectral cameras can monitor crop health, allowing farmers to identify areas needing irrigation or fertilization. This reduces water and fertilizer use, leading to increased yields and decreased environmental impact.
- Vertical Farming: Vertical farms utilize stacked layers in controlled environments, enabling year-round crop production in urban areas. These systems often use hydroponics or aeroponics, reducing water consumption and eliminating the need for pesticides.
- Alternative Protein Sources: The development of alternative protein sources, such as plant-based meats and cultivated meat, offers a more sustainable alternative to traditional animal agriculture. These options typically require fewer resources and produce fewer greenhouse gas emissions.
3D Printing in Food Production
D printing, also known as additive manufacturing, is making its mark in food production. This technology allows for the creation of complex food structures and customized products, opening up new possibilities for culinary creativity and personalized nutrition.
- Food Customization: 3D printing allows for the creation of foods with unique shapes, textures, and flavors. Chefs and food scientists can design intricate dishes and tailor food products to specific dietary needs or preferences. For instance, personalized meals for individuals with allergies or specific nutritional requirements can be easily created.
- Waste Reduction: 3D printing can use food waste as a raw material, transforming it into edible products. This helps to reduce food waste and promote circular economy principles. For example, food scraps from fruits and vegetables can be repurposed into flavorful snacks or ingredients.
- Novel Food Textures: 3D printing can create foods with innovative textures and mouthfeels. This technology can precisely control the layering and composition of food materials, resulting in unique culinary experiences.
Genetically Modified Foods and Global Food Security
Genetically modified (GM) foods, also known as genetically engineered (GE) foods, have the potential to significantly impact global food security. By altering the genetic makeup of crops, scientists can enhance their nutritional value, increase yields, and improve their resistance to pests and diseases.
- Increased Crop Yields: GM crops, such as Bt corn and herbicide-resistant soybeans, can produce higher yields, which can help to feed a growing global population. Increased yields can lead to lower food prices and greater food availability.
- Enhanced Nutritional Value: Some GM crops are engineered to have enhanced nutritional value. For example, Golden Rice is genetically modified to produce beta-carotene, a precursor to vitamin A, which can help to combat vitamin A deficiency in developing countries.
- Pest and Disease Resistance: GM crops can be engineered to resist pests and diseases, reducing the need for pesticides and herbicides. This can lead to lower production costs and reduced environmental impact. For instance, crops resistant to certain insects, such as the European corn borer, require less pesticide application.
Food Pairings: Harmony on the Plate
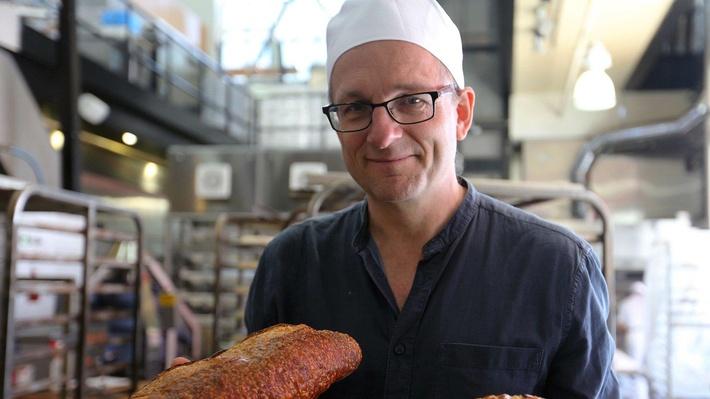
The art of food pairing elevates a meal from simply satisfying hunger to a multi-sensory experience. By understanding the chemical compounds within ingredients and how they interact, chefs and food enthusiasts can create combinations that highlight flavors, textures, and aromas, resulting in a more enjoyable and memorable dining experience. The principles behind these pairings are rooted in scientific understanding, allowing for a level of precision that transforms cooking into an art form.
Principles of Flavor Pairing
Flavor pairing relies on the concept that certain flavors share common volatile aroma compounds. When these compounds are present in two or more ingredients, they can create a synergistic effect, where the combined flavor is greater than the sum of its parts. This is often referred to as flavor bridging. Several key principles guide successful flavor pairings:
- Common Aroma Compounds: Ingredients that share a significant number of aroma compounds are more likely to pair well. For instance, chocolate and coffee both contain volatile compounds such as pyrazines, which contribute to their shared roasted, nutty notes.
- Flavor Profiles: Understanding the overall flavor profile of an ingredient (e.g., sweet, sour, bitter, salty, umami) is crucial. Pairing complementary flavors or balancing contrasting ones is a common technique.
- Texture and Mouthfeel: Texture plays a vital role in the dining experience. Pairing ingredients with contrasting textures can create a more interesting and balanced dish. Consider the crispness of a fried element against the creaminess of a sauce.
- Regional and Cultural Influences: Traditional food pairings often evolve over time and are influenced by regional availability of ingredients and cultural preferences. These pairings often represent a history of successful combinations.
The flavor wheel, a visual representation of flavor profiles, is a valuable tool for identifying potential pairings based on shared characteristics.
Classic Food and Wine Pairings
Wine and food pairings are a well-established practice that enhances both the food and the wine. The goal is to find a balance where neither the food nor the wine overpowers the other. Several classic pairings have become iconic due to their ability to create a harmonious balance of flavors.
- Red Wine and Red Meat: The tannins in red wine bind with the proteins in red meat, softening the wine’s astringency and enhancing the meat’s richness. Examples include Cabernet Sauvignon with grilled steak, or Merlot with roasted lamb.
- White Wine and Seafood: The acidity in white wine cuts through the richness of seafood, cleansing the palate and complementing the delicate flavors. Chardonnay pairs well with lobster, and Sauvignon Blanc complements oysters.
- Sweet Wine and Dessert: Sweet wines, such as Sauternes or late-harvest Riesling, provide a counterpoint to the sweetness of desserts, preventing them from becoming cloying. They can also mirror the flavors of the dessert, creating a harmonious combination.
- Sparkling Wine and Fried Foods: The effervescence and acidity of sparkling wine cut through the oiliness of fried foods, refreshing the palate. Champagne pairs well with fried chicken or French fries.
Tasting Menu: Contrasting Flavors and Textures
Designing a tasting menu that showcases contrasting flavors and textures requires careful consideration of each element’s characteristics and how they will interact. This menu aims to create a dynamic and engaging dining experience, where each course builds upon the previous one.
- Amuse-bouche: A single oyster with a mignonette sauce. The briny, fresh oyster is contrasted by the acidic and slightly sweet mignonette, providing a clean and refreshing start.
- Appetizer: Seared scallops with cauliflower purée and crispy pancetta. The sweetness of the scallops is balanced by the earthy cauliflower and the salty, crunchy pancetta, offering a range of textures.
- Soup: Creamy tomato soup with a grilled cheese crouton. The rich, smooth soup is complemented by the crispy, cheesy crouton, creating a textural contrast and familiar flavor combination.
- Main Course: Pan-seared duck breast with cherry sauce, served with potato gratin and asparagus. The rich duck breast is complemented by the sweet and tart cherry sauce, while the creamy potato gratin and tender asparagus provide textural variation.
- Dessert: Chocolate lava cake with raspberry coulis and vanilla ice cream. The warm, gooey chocolate cake is contrasted by the tart raspberry coulis and the cold, creamy ice cream, offering a perfect balance of textures and flavors.
- Wine Pairings (examples):
- Oyster with Mignonette: Dry, crisp Champagne or a dry, crisp Sauvignon Blanc.
- Seared Scallops: A crisp, dry white wine like a Chablis or a light-bodied Chardonnay.
- Tomato Soup: A medium-bodied red wine like a Pinot Noir.
- Duck Breast: A bold red wine like a Cabernet Sauvignon or a Pinot Noir.
- Chocolate Lava Cake: A sweet wine like a Tawny Port or a late-harvest Zinfandel.
The Science of Baking: Chemistry in Action
Baking, often perceived as an art, is fundamentally a science. It’s a realm where chemical reactions, precise measurements, and controlled environments converge to transform simple ingredients into delectable creations. Understanding the underlying principles of baking, from the role of gluten to the impact of flour types, allows bakers to consistently achieve desired results and troubleshoot potential problems.
The Role of Gluten in Bread Making
Gluten, a protein complex formed from glutenin and gliadin found in wheat flour, is the structural backbone of bread. Its development is crucial for trapping the carbon dioxide produced by yeast fermentation, which causes the dough to rise and develop its characteristic airy texture.The process of gluten formation involves the following key elements:
- Hydration: When flour is mixed with water, glutenin and gliadin proteins hydrate and begin to interact.
- Kneading: Kneading the dough physically aligns and strengthens the gluten strands, creating a network.
- Fermentation: During fermentation, yeast consumes sugars and produces carbon dioxide, which is trapped by the gluten network, causing the dough to expand.
- Baking: Heat from the oven sets the gluten structure, solidifying the bread’s shape. The starch gelatinizes, and the Maillard reaction (browning) occurs on the crust.
The strength of the gluten network determines the bread’s texture. A strong gluten network results in a chewy bread, while a weaker network produces a softer loaf. Over-kneading can lead to a tough bread, whereas under-kneading can result in a dense, flat loaf. The amount of gluten present varies depending on the flour type; bread flour, for example, has a higher protein content (and therefore more gluten) than cake flour.
Procedure for Making Perfect Sourdough Bread
Sourdough bread, with its characteristic tangy flavor and complex texture, relies on a naturally occurring leavening agent: a sourdough starter. This starter is a culture of wild yeasts and lactic acid bacteria that ferment the flour and water mixture. Making sourdough requires patience and precision.The following steps Artikel a procedure for making a perfect sourdough bread:
- Maintaining the Starter: Feed your sourdough starter regularly (typically once or twice a day) with equal parts flour and water to keep it active and bubbly. A healthy starter should double in size within a few hours after feeding.
- Autolyse: Combine the flour and water in a bowl and let it rest for 30-60 minutes. This allows the flour to fully hydrate, which helps develop gluten.
- Mixing: Add the starter and salt to the autolysed dough. Mix until everything is incorporated.
- Bulk Fermentation: Allow the dough to rise at room temperature for 4-6 hours, performing stretch-and-folds every 30-60 minutes for the first few hours. Stretch-and-folds help strengthen the gluten and redistribute the yeast and bacteria.
- Shaping: Gently shape the dough into a round or oblong loaf.
- Proofing: Place the shaped dough in a banneton basket (or a bowl lined with a floured cloth) and refrigerate overnight (12-18 hours). This slow, cold proofing develops flavor and improves the crust.
- Baking: Preheat your oven with a Dutch oven inside to 450°F (232°C). Carefully place the proofed dough into the hot Dutch oven, score the top with a sharp knife or lame, and bake covered for 20 minutes. Remove the lid and bake for another 25-30 minutes, or until the crust is deeply golden brown.
- Cooling: Allow the bread to cool completely on a wire rack before slicing and enjoying. This prevents a gummy texture.
The timing of each step is critical. Factors like ambient temperature and the activity of your starter will influence the fermentation time. The baker’s understanding of these factors allows for adjustments to achieve the desired outcome.
How the Type of Flour Affects the Final Product
The type of flour used in baking significantly impacts the bread’s final characteristics, including its texture, rise, and flavor. Different flours have varying protein contents, ash contents, and particle sizes, all of which influence the baking process and the resulting bread.Here’s how different flour types affect bread:
- Bread Flour: Bread flour, with its high protein content (around 12-14%), develops a strong gluten network, resulting in a chewy texture and a good rise. It’s ideal for crusty breads like baguettes and sourdough.
- All-Purpose Flour: All-purpose flour has a moderate protein content (around 10-12%), making it versatile. It can be used for a variety of baked goods, including bread, cookies, and cakes.
- Cake Flour: Cake flour has a low protein content (around 8-10%), producing a delicate and tender crumb. It is typically used for cakes and pastries.
- Whole Wheat Flour: Whole wheat flour contains the entire wheat kernel, including the bran and germ. It has a higher fiber content and a nutty flavor. Bread made with whole wheat flour tends to be denser than bread made with white flour. The bran can interfere with gluten development, so it is often combined with bread flour.
- Rye Flour: Rye flour contributes a distinct flavor and texture to bread. It has a lower gluten content than wheat flour, often resulting in a denser loaf. Rye flour is often used in combination with wheat flour.
The ash content of flour also affects the bread’s characteristics. Ash refers to the mineral content of the flour. Higher ash content can result in a darker crust and a slightly more complex flavor. The particle size of the flour influences how it absorbs water and how the gluten develops. Finer flours generally absorb water more quickly.
The baker must consider these factors when selecting flour and adjusting the recipe to achieve the desired outcome. For example, when substituting whole wheat flour for all-purpose flour, it may be necessary to increase the hydration to compensate for the higher absorption rate of the whole wheat flour.
Wrap-Up
In conclusion, the exploration of food a delicious science unveils the fascinating intersection of culinary arts and scientific principles. From understanding the Maillard reaction to the intricacies of food preservation and the promise of future food innovations, we’ve journeyed through the essential elements that make food both delicious and a subject of scientific inquiry. By appreciating the science behind our meals, we can enhance our culinary skills and appreciate the complex world of food in a deeper, more meaningful way.