Food chemistry textbook delves into the fascinating world where science meets sustenance. This comprehensive resource explores the fundamental principles that govern the composition, properties, and transformations of food. From understanding the intricate structures of carbohydrates and proteins to unraveling the mysteries of flavors and aromas, this book offers a deep dive into the molecular interactions that shape our culinary experiences.
We will embark on a journey through the building blocks of food, exploring how they interact during processing, storage, and consumption.
The journey begins with an introduction to the core concepts of food chemistry, setting the stage for detailed examinations of key components like water, carbohydrates, lipids, and proteins. Each chapter will provide insights into the role these components play in food texture, flavor, and stability. The textbook will then venture into the realms of enzymes, food colors, and flavor compounds, providing a detailed look at food additives, preservatives, and various processing techniques.
Moreover, it covers the essential aspects of food packaging, storage, safety, and quality control, with a focus on nutritional aspects and advanced topics, including the impact of food chemistry on sustainability and food security.
Introduction to Food Chemistry
Food chemistry is a scientific discipline that explores the chemical processes and interactions of all biological and non-biological components of foods. It is a vital field, essential for understanding the composition, properties, and changes foods undergo during processing, storage, and consumption. The knowledge gained from food chemistry is critical in developing safer, more nutritious, and palatable food products.
Fundamental Principles and Significance
Food chemistry is built upon several fundamental principles. These include understanding the molecular structure of food components, such as carbohydrates, proteins, lipids, vitamins, and minerals; investigating the chemical reactions that occur within food systems; and examining the impact of processing and storage conditions on food quality and safety. The significance of food chemistry lies in its ability to:
- Improve food quality and safety: By understanding the chemical reactions that cause food spoilage, food chemists can develop methods to prevent or slow down these reactions, extending shelf life and ensuring food safety.
- Enhance nutritional value: Food chemistry plays a crucial role in understanding how nutrients are affected by processing and storage. This knowledge helps in optimizing food processing techniques to retain or enhance the nutritional value of foods.
- Develop new food products: Food chemists use their knowledge to create new food products with improved flavor, texture, and nutritional profiles. This includes the development of functional foods and novel food ingredients.
- Understand food sensory properties: The chemical composition of food directly influences its sensory properties, such as taste, aroma, and texture. Food chemists investigate these relationships to improve the consumer experience.
- Ensure food authenticity: Food chemistry provides tools to detect food fraud and adulteration, ensuring that consumers receive the products they expect.
Brief History of Food Chemistry, Food chemistry textbook
The study of food chemistry has evolved over centuries, with its roots in early observations of food preservation and preparation. Significant milestones in its development include:
- Early Observations: Ancient civilizations practiced food preservation techniques such as salting, smoking, and fermentation, though the underlying chemical principles were not understood.
- 18th and 19th Centuries: The rise of chemistry as a scientific discipline provided the foundation for food chemistry. Scientists began to analyze the composition of foods, identifying key components like carbohydrates, proteins, and fats. Key figures like Antoine Lavoisier contributed to understanding the role of oxygen in food spoilage.
- Early 20th Century: The development of analytical techniques, such as chromatography and spectroscopy, allowed for more detailed analysis of food components. The impact of heat treatment on food safety and preservation, such as pasteurization, became better understood.
- Mid-20th Century to Present: Advances in biochemistry, molecular biology, and food processing technologies have greatly expanded the scope of food chemistry. The understanding of food additives, food allergens, and the impact of processing on nutritional value has improved. Food chemistry has become increasingly interdisciplinary, integrating with fields like nutrition, toxicology, and sensory science.
Scope and Relationship to Other Fields
Food chemistry is a broad field, encompassing many areas of study and interacting with other scientific disciplines. Its scope includes:
- Food Composition: This involves the analysis of the major and minor components of food, including water, carbohydrates, proteins, lipids, vitamins, minerals, and other bioactive compounds.
- Food Processing: This focuses on the chemical changes that occur during food processing techniques such as heating, cooling, drying, fermentation, and extrusion.
- Food Preservation: This area studies methods to extend the shelf life of foods by inhibiting microbial growth and chemical reactions that cause spoilage.
- Food Analysis: This involves the development and application of analytical techniques to determine the composition, quality, and safety of food products.
- Food Safety: This field examines the chemical and biological hazards in food and develops strategies to minimize the risks of foodborne illnesses.
- Food Packaging: This studies the interaction between food and packaging materials, focusing on the impact of packaging on food quality and safety.
Food chemistry is closely related to several other fields:
- Nutrition: Food chemistry provides the foundation for understanding the nutritional value of foods and how they interact with the human body.
- Food Microbiology: This field studies the microorganisms that affect food, including spoilage organisms and pathogens.
- Food Engineering: This discipline applies engineering principles to food processing and manufacturing.
- Toxicology: Food chemistry is essential for understanding the toxicological effects of food components and contaminants.
- Sensory Science: This field investigates the sensory properties of food, such as taste, aroma, and texture, and their relationship to food chemistry.
Water in Food Systems
Water is a fundamental component of nearly all food products, playing a crucial role in determining their texture, appearance, stability, and shelf life. Understanding the properties and behavior of water within food matrices is essential for food scientists and technologists to control and predict the characteristics of food products. Its influence extends from basic taste and palatability to complex chemical reactions and microbial growth, making it a central consideration in food processing and preservation.
Role of Water in Food: Properties and Functions
Water’s unique properties arise from its polar nature, which allows it to form hydrogen bonds. This characteristic significantly influences its behavior in food systems.
- Solvent: Water acts as a universal solvent, dissolving a wide range of food components, including sugars, salts, vitamins, and some proteins. This solvent capability facilitates the transport of nutrients and flavors throughout the food matrix. For instance, in fruit juices, water dissolves sugars and acids, contributing to their characteristic taste.
- Reactant: Water participates in various chemical reactions, such as hydrolysis, where it breaks down complex molecules into simpler ones. This is important in the ripening of fruits, where starch is broken down into sugars by hydrolysis.
- Plasticizer: Water acts as a plasticizer, increasing the flexibility and mobility of food components. It affects the texture of foods; for example, in bread, water hydrates gluten proteins, forming a network that gives the bread its structure and chewiness.
- Heat Transfer Medium: Water is an excellent heat transfer medium, facilitating cooking and preservation processes like blanching and pasteurization. During cooking, water transfers heat to the food, causing changes in texture and flavor.
- Texture and Structure: Water contributes significantly to the texture and structure of foods. The presence of water affects the crispness of vegetables, the softness of cakes, and the viscosity of sauces. The structure of ice cream, for example, is dependent on the formation of ice crystals.
Different Forms of Water in Food and Impact on Food Quality
Water in food exists in different forms, each affecting food quality differently. These forms include free water, bound water, and immobilized water.
- Free Water: This is water that is not bound to food components and is readily available for microbial growth and chemical reactions. It contributes to the juiciness and texture of foods. Its presence in high amounts leads to rapid spoilage. For example, in fresh fruits and vegetables, free water is abundant, making them susceptible to microbial spoilage if not properly stored.
- Bound Water: This water is tightly associated with food components through hydrogen bonds or other interactions. It is not available for microbial growth or most chemical reactions. Bound water contributes to the stability of food. For example, in dried foods, the water is largely bound, which contributes to the food’s long shelf life.
- Immobilized Water: This water is physically entrapped within the food matrix and exhibits intermediate properties between free and bound water. It may be less available than free water but can still support some microbial growth. The water in the pores of a sponge cake represents immobilized water.
The relative proportions of these water forms determine a food’s shelf life, texture, and overall quality. Foods with a high proportion of free water tend to spoil more quickly, while those with a higher proportion of bound water are generally more stable.
Water Activity and its Influence on Food Preservation
Water activity (aw) is a measure of the amount of unbound or “free” water available in a food system for chemical reactions and microbial growth. It is defined as the ratio of the water vapor pressure of the food to the vapor pressure of pure water at the same temperature. Water activity is a critical factor in food preservation.
aw = P / P₀
where:
- aw = water activity
- P = vapor pressure of water in the food
- P₀ = vapor pressure of pure water
Lowering water activity through methods like drying, freezing, or adding solutes (e.g., sugar or salt) is a common strategy to inhibit microbial growth and extend shelf life. The minimum water activity required for the growth of most bacteria is around 0.90, for yeasts around 0.80, and for molds around 0.70.
Effects of Water Activity on Food
Water Activity (aw) Range | Microbial Growth | Chemical Reactions | Physical Changes |
---|---|---|---|
> 0.95 | Rapid growth of bacteria, yeasts, and molds. | Rapid enzymatic and non-enzymatic reactions (e.g., lipid oxidation, Maillard browning). | Loss of texture, softening, spoilage. |
0.90 – 0.95 | Growth of most bacteria and some yeasts and molds. | Accelerated enzymatic and non-enzymatic reactions. | Changes in texture and appearance. |
0.80 – 0.90 | Inhibition of most bacteria; some yeasts and molds can still grow. | Slower reaction rates, but still significant. | Some changes in texture and appearance; may become firm. |
< 0.80 | Inhibition of most microbial growth; some xerophilic molds and osmophilic yeasts can still grow. | Significantly reduced reaction rates. | Increased stability; may become brittle or hard. |
Carbohydrates in Food
Carbohydrates are a fundamental class of organic molecules, serving as a primary energy source and structural component in food. Their diverse structures and reactivity play a crucial role in determining the characteristics of food products, influencing texture, flavor, color, and overall quality. Understanding the properties and reactions of carbohydrates is essential for food scientists and anyone involved in food processing and preparation.
Classification and Structure of Carbohydrates
Carbohydrates are broadly classified based on the number of sugar units they contain. These sugar units, or monosaccharides, are the simplest carbohydrates.
- Monosaccharides: These are simple sugars, such as glucose, fructose, and galactose. They cannot be broken down into smaller carbohydrate units. The basic formula is (CH₂O)n, where ‘n’ typically ranges from 3 to 7.
- Glucose: Also known as dextrose, glucose is a hexose (6-carbon) sugar and is the primary energy source for cells. It exists in both linear and cyclic forms.
- Fructose: Another hexose, fructose is the sweetest naturally occurring sugar. It is commonly found in fruits and honey. Its structure differs from glucose, which accounts for its higher sweetness.
- Galactose: A hexose sugar that is less sweet than glucose or fructose. It is a component of lactose (milk sugar).
- Disaccharides: These are formed by the glycosidic linkage of two monosaccharides. Common examples include sucrose, lactose, and maltose.
- Sucrose: Commonly known as table sugar, sucrose is a disaccharide composed of glucose and fructose.
- Lactose: Found in milk, lactose is composed of glucose and galactose.
- Maltose: Maltose is formed from two glucose molecules and is produced during the breakdown of starch.
- Polysaccharides: These are complex carbohydrates composed of long chains of monosaccharides linked together. They serve as energy storage molecules (e.g., starch in plants, glycogen in animals) and structural components (e.g., cellulose in plants).
- Starch: A major storage polysaccharide in plants, starch is composed of amylose (linear chains of glucose) and amylopectin (branched chains of glucose).
- Cellulose: A structural polysaccharide found in plant cell walls, cellulose is composed of long, linear chains of glucose. Humans cannot digest cellulose.
- Glycogen: The storage form of glucose in animals, glycogen is highly branched and stored primarily in the liver and muscles.
Reactions of Carbohydrates in Food Processing
Carbohydrates undergo various reactions during food processing, influencing the final product’s characteristics. These reactions are often affected by factors such as temperature, pH, and the presence of other food components.
- Monosaccharides:
- Caramelization: At high temperatures, monosaccharides undergo caramelization, a non-enzymatic browning reaction that produces a complex mixture of volatile and non-volatile compounds, contributing to color, flavor, and aroma development. For example, the browning of sugar in candy making is due to caramelization.
- Fermentation: Monosaccharides are readily fermented by microorganisms, such as yeasts and bacteria, to produce ethanol, carbon dioxide, and other byproducts. This process is used in the production of bread, beer, and wine.
- Disaccharides:
- Hydrolysis: Disaccharides can be hydrolyzed (broken down) into their constituent monosaccharides in the presence of water and an acid or enzyme. For instance, the hydrolysis of sucrose yields glucose and fructose.
- Maillard Reaction: Disaccharides, like sucrose, can participate in the Maillard reaction, although less readily than monosaccharides. The hydrolysis of sucrose to glucose and fructose can increase the rate of the Maillard reaction.
- Polysaccharides:
- Gelatinization: Starch granules absorb water and swell when heated in the presence of water, leading to gelatinization. This process is essential in thickening sauces and gravies.
- Dextrinization: When starch is heated in the absence of water, it breaks down into smaller polysaccharides called dextrins, resulting in a change in color and flavor. This is observed when toasting bread.
- Acid Hydrolysis: Polysaccharides can be broken down into smaller units (dextrins, maltose, glucose) by acid hydrolysis, impacting the texture and viscosity of food products.
Role of Carbohydrates in Food Texture, Flavor, and Color
Carbohydrates significantly impact the sensory properties of food, contributing to texture, flavor, and color development.
- Texture:
- Starch: The gelatinization of starch provides thickening and texture in sauces, gravies, and puddings. The ratio of amylose and amylopectin in starch influences the texture, with higher amylose content leading to firmer gels.
- Sugars: Sugars contribute to the chewiness, tenderness, and spread of baked goods. The crystallization of sugars in candies determines their texture.
- Polysaccharides (e.g., gums): These can be added to food products to modify texture by thickening, gelling, or stabilizing.
- Flavor:
- Sugars: Monosaccharides and disaccharides provide sweetness. Fructose is sweeter than glucose, and sucrose is sweeter than lactose.
- Caramelization products: The caramelization of sugars produces a wide range of flavor compounds, contributing to the characteristic flavors of caramel, toffee, and other confections.
- Maillard reaction products: The Maillard reaction produces numerous flavor compounds, responsible for the savory flavors of cooked meats, baked bread, and roasted coffee.
- Color:
- Caramelization: This reaction is responsible for the brown color development in caramel and toffee.
- Maillard reaction: The Maillard reaction is a major contributor to browning in cooked foods, such as bread crust, roasted meats, and coffee beans.
- Dextrinization: Dextrinization of starch contributes to the golden-brown color of toasted bread.
Maillard Reaction
The Maillard reaction is a complex chemical reaction between reducing sugars (such as glucose and fructose) and amino acids, producing a wide range of flavor and color compounds. This non-enzymatic browning reaction is crucial in the flavor and color development of many foods.
- Steps of the Maillard Reaction:
- Initial Stage: The carbonyl group of a reducing sugar reacts with the amino group of an amino acid or protein, forming a glycosylamine. This unstable compound then undergoes an Amadori rearrangement, producing a more stable ketoamine.
- Intermediate Stage: The ketoamine undergoes further reactions, including dehydration, degradation, and fragmentation, forming a variety of intermediate compounds. These include reductones, diacetyl, and furans.
- Advanced Stage: The intermediate compounds polymerize and react further, forming complex melanoidins, which are responsible for the brown color and contribute to the flavor and aroma of the food.
- Factors Influencing the Maillard Reaction:
- Temperature: Higher temperatures accelerate the reaction rate. The reaction typically occurs at temperatures above 120°C (248°F), but it can proceed slowly at lower temperatures over extended periods.
- pH: The reaction is favored at alkaline pH levels.
- Water activity: An optimal water activity (aw) range is needed for the reaction to occur. Too much or too little water can inhibit the reaction.
- Reducing sugars: The type and concentration of reducing sugars (glucose, fructose) affect the reaction rate and the flavor compounds produced.
- Amino acids: The type and concentration of amino acids influence the flavor and aroma profiles. Different amino acids yield different flavor compounds.
- Presence of catalysts: Certain catalysts, such as metal ions, can accelerate the Maillard reaction.
Lipids in Food
Lipids, commonly known as fats and oils, are essential macronutrients in the human diet and play a crucial role in food science and technology. They contribute significantly to the sensory properties of food, influencing flavor, texture, and appearance. Furthermore, lipids are important for nutrient absorption and energy storage. Understanding the structure, properties, and behavior of lipids is fundamental to food chemistry.
Structure and Properties of Lipids
Lipids are a diverse group of organic molecules characterized by their insolubility in water and solubility in nonpolar solvents. Their basic structure and properties dictate their behavior in food systems.* Lipids are primarily composed of carbon, hydrogen, and oxygen atoms.
The most common type of lipid found in food is triglycerides, which are composed of a glycerol molecule (a three-carbon alcohol) bonded to three fatty acids via ester linkages.
- Fatty acids are long hydrocarbon chains with a carboxyl group (-COOH) at one end.
- The length and saturation of the fatty acid chains determine the physical properties of the lipid.
Shorter chain fatty acids generally have lower melting points.
- Saturation refers to the presence of single bonds between carbon atoms. Saturated fatty acids have no double bonds, while unsaturated fatty acids have one or more double bonds.
- Other important lipid classes include phospholipids, which are important components of cell membranes and emulsifiers in food, and sterols, such as cholesterol.
- Lipids provide a concentrated source of energy, with approximately 9 kcal (37 kJ) per gram.
Saturated, Unsaturated, and Trans Fats
The structure of fatty acids significantly influences their properties and health implications. The degree of saturation, the presence of double bonds, and the configuration around these bonds differentiate the various types of fats.* Saturated Fats: These fats contain no carbon-carbon double bonds.
They are typically solid at room temperature.
Examples include butter, lard, and coconut oil.
Saturated fats are associated with increased levels of LDL (“bad”) cholesterol, potentially increasing the risk of cardiovascular disease.
Unsaturated Fats
These fats contain one or more carbon-carbon double bonds.
They are typically liquid at room temperature.
They are further categorized into monounsaturated and polyunsaturated fats.
Monounsaturated Fats
Contain one double bond. Examples include olive oil and avocados.
Polyunsaturated Fats
Contain two or more double bonds. Examples include soybean oil, corn oil, and fish oil. Unsaturated fats are generally considered healthier than saturated fats, as they can help lower LDL cholesterol levels.
Trans Fats
These fats are formed during the process of hydrogenation, where hydrogen is added to unsaturated fats to make them more solid.
Trans fats contain trans double bonds, which give them a different shape compared to the cis double bonds found in naturally occurring unsaturated fats.
Trans fats are associated with increased LDL cholesterol levels and decreased HDL (“good”) cholesterol levels, posing a significant risk to cardiovascular health.
Examples include partially hydrogenated vegetable oils, which were once commonly used in processed foods.
Many countries and regions have restricted or banned the use of trans fats in food production.
Role of Lipids in Food Flavor, Texture, and Stability
Lipids play a vital role in the sensory characteristics and shelf life of food products. They contribute significantly to the overall quality and consumer acceptance of food.* Flavor: Lipids can dissolve and carry flavor compounds, enhancing the perception of flavor. They also contribute their own flavors, such as the buttery flavor of butter or the nutty flavor of olive oil.
Lipids act as a solvent for fat-soluble flavor compounds, amplifying their impact.
The Maillard reaction, which occurs during cooking, can involve lipids, leading to the formation of desirable flavor compounds.
Texture
Lipids influence the texture of food products in several ways.
They contribute to the tenderness of baked goods by interfering with gluten development.
They provide a smooth, creamy mouthfeel in products like ice cream and sauces.
They can create a crispy texture in fried foods.
Stability
Lipids can affect the stability of food products.
They act as a barrier to moisture, preventing food from drying out.
They can stabilize emulsions, such as mayonnaise, preventing separation.
However, lipids are susceptible to oxidation, which can lead to rancidity and off-flavors, decreasing shelf life.
Lipid Oxidation Reactions
Lipid oxidation is a major cause of food spoilage, leading to rancidity and the development of undesirable flavors and odors. Understanding the mechanisms of lipid oxidation is critical for preserving food quality.* Lipid oxidation is a free radical chain reaction.
It is initiated by the abstraction of a hydrogen atom from a fatty acid molecule, typically at a carbon atom adjacent to a double bond (allylic position).
This produces a free radical, which reacts with oxygen to form a peroxy radical.
The peroxy radical then abstracts a hydrogen atom from another fatty acid molecule, propagating the chain reaction.
Initiation
This stage involves the formation of free radicals.
Free radicals can be generated by
Exposure to light (photoxidation).
– Heat.
The presence of metal ions (e.g., iron, copper).
Enzymes (e.g., lipoxygenases).
Propagation
This stage involves the chain reaction of free radicals.
Peroxy radicals react with other fatty acids, forming hydroperoxides.
Hydroperoxides are unstable and can decompose into a variety of volatile compounds.
Termination
This stage involves the removal of free radicals.
Antioxidants, such as vitamin E and vitamin C, can donate hydrogen atoms to free radicals, terminating the chain reaction.
Free radicals can also react with each other, forming stable, non-radical products.
Consequences of Lipid Oxidation
Rancidity
The development of unpleasant flavors and odors.
Loss of Nutritional Value
Oxidation can destroy essential fatty acids and fat-soluble vitamins.
Color Changes
Oxidation can lead to discoloration of the food.
Texture Changes
Oxidation can cause changes in the texture of the food.
Formation of Toxic Compounds
Lipid oxidation can generate potentially harmful compounds.
Factors Affecting Lipid Oxidation
Degree of Unsaturation
Polyunsaturated fatty acids are more susceptible to oxidation than saturated fatty acids.
Temperature
Higher temperatures accelerate oxidation.
Presence of Oxygen
Oxygen is essential for lipid oxidation.
Presence of Pro-oxidants
Metal ions, light, and certain enzymes can promote oxidation.
Presence of Antioxidants
Discover the crucial elements that make aldi vs whole foods the top choice.
Antioxidants can inhibit oxidation.
Preventing Lipid Oxidation
Use of Antioxidants
Adding antioxidants to food products.
Control of Oxygen
Packaging food in airtight containers or using modified atmosphere packaging.
Control of Temperature
Storing food at low temperatures.
Control of Light
Protecting food from light exposure.
Use of Chelating Agents
Removing metal ions that can catalyze oxidation.
Proteins in Food
Proteins are essential macronutrients in food, playing a critical role in both nutritional value and functional properties. They are complex molecules composed of amino acids linked by peptide bonds. Understanding the structure and behavior of proteins is crucial for comprehending various aspects of food chemistry, from the nutritional quality of food products to their textural characteristics and processing behavior. This section explores the structure, function, and impact of proteins in food systems.
Structure and Function of Proteins
Proteins are the workhorses of the food system, performing diverse functions essential for both nutritional value and food processing. They are constructed from amino acids, which are linked together by peptide bonds to form polypeptide chains. These chains fold into complex three-dimensional structures, giving each protein its unique function.The primary structure of a protein is its amino acid sequence. The secondary structure involves local folding patterns, such as alpha-helices and beta-sheets, stabilized by hydrogen bonds.
The tertiary structure describes the overall three-dimensional shape of a single polypeptide chain, resulting from interactions between the amino acid side chains, including hydrophobic interactions, disulfide bridges, and ionic bonds. Finally, the quaternary structure arises when multiple polypeptide chains (subunits) associate to form a functional protein complex. The specific amino acid sequence and the resulting three-dimensional structure dictate a protein’s function.Proteins perform numerous vital roles in food:
- Enzymes: Catalyze biochemical reactions, such as the breakdown of carbohydrates or the formation of flavors.
- Structural components: Contribute to the texture and physical properties of food, such as the formation of gluten in bread.
- Transport: Carry molecules within food systems.
- Storage: Provide a source of amino acids.
- Nutritional value: Provide essential amino acids that the human body cannot synthesize.
Denaturation and Coagulation of Proteins
Food processing often involves changes in temperature, pH, and the presence of other chemicals, which can affect protein structure and function. Denaturation and coagulation are two key processes that proteins undergo during food processing. Denaturation is the process where a protein loses its native three-dimensional structure, typically without breaking peptide bonds. This unfolding can be caused by heat, changes in pH, the addition of organic solvents, or the presence of heavy metals.
Denaturation disrupts the weaker bonds (hydrogen bonds, hydrophobic interactions, etc.) that maintain the protein’s three-dimensional shape, leading to unfolding of the protein. Denaturation does not necessarily change the primary structure of the protein, but it alters its physical and chemical properties. Coagulation is the aggregation or clumping together of denatured proteins. This often results in a change in the physical state of the food, such as the setting of egg whites upon heating or the formation of curds in milk during cheese making.
Denatured proteins expose hydrophobic regions that were previously buried within the folded structure. These exposed regions can then interact with each other, forming a network that traps water molecules and other food components, leading to a change in texture and appearance. The rate and extent of coagulation depend on factors such as temperature, pH, and the concentration of the protein.
Role of Proteins in Food Texture, Emulsification, and Foaming
Proteins are critical for achieving desirable textures, stabilizing emulsions, and creating foams in various food products. Their unique structural properties and interactions with other food components enable these functionalities.Proteins significantly influence food texture:
- Gluten in bread: Gluten proteins (gliadin and glutenin) form a network that traps carbon dioxide produced by yeast, causing the dough to rise and providing the characteristic chewy texture of bread.
- Meat: Proteins like collagen and myosin contribute to the texture of meat products. Collagen provides structural support, while myosin is responsible for muscle contraction and contributes to the texture after cooking.
- Gelatin: Derived from collagen, gelatin forms gels in products like jellies and marshmallows, providing a soft, yielding texture.
Proteins are effective emulsifiers, meaning they can stabilize mixtures of oil and water, preventing them from separating:
- Milk proteins (caseins): Caseins in milk surround fat droplets, preventing them from coalescing and forming a stable emulsion. This is why milk appears white.
- Egg proteins: Egg yolk contains lipoproteins that act as emulsifiers in products like mayonnaise and salad dressings.
Proteins are crucial for foaming, creating air-filled structures that give volume and lightness to food:
- Egg whites: When egg whites are whipped, proteins denature and unfold, allowing them to surround air bubbles and form a stable foam. This is essential in meringues, soufflés, and angel food cake.
- Whipped cream: Proteins in cream, primarily caseins and whey proteins, denature and trap air bubbles, creating a stable foam.
Protein Sources and Applications in Food
Various protein sources are utilized in food processing, each offering unique functional properties and nutritional profiles.
Protein Source | Protein Type | Applications | Key Functional Properties |
---|---|---|---|
Milk | Casein, Whey Proteins | Cheese, yogurt, ice cream, protein supplements, infant formula | Emulsification, foaming, gelation, water binding, nutritional value |
Eggs | Ovalbumin, Conalbumin, Ovomucoid | Baked goods, sauces, custards, meringues, emulsified products (mayonnaise) | Foaming, emulsification, coagulation, texture, flavor, color |
Soybeans | Glycinin, β-conglycinin | Tofu, soy milk, textured vegetable protein (TVP), meat analogs | Texturization, emulsification, water binding, fat absorption |
Wheat | Glutenin, Gliadin | Bread, pasta, baked goods | Viscoelasticity, structure formation, dough development |
Enzymes in Food
Enzymes are biological catalysts, primarily proteins, that accelerate biochemical reactions within food systems. Their presence significantly impacts food quality, affecting flavor, texture, appearance, and nutritional value. Understanding enzyme behavior is crucial for both food preservation and the production of desirable food characteristics.
Nature and Role of Enzymes in Food Systems
Enzymes are highly specific, acting on particular substrates to facilitate specific reactions. This specificity is due to the unique three-dimensional structure of the enzyme, which allows it to bind to the substrate at its active site. This interaction lowers the activation energy of the reaction, thereby speeding it up. The role of enzymes in food can be either beneficial or detrimental.
For instance, enzymes can be used to ripen fruits or tenderize meat, but they can also cause undesirable changes like browning or off-flavors.
Factors Affecting Enzyme Activity
Several factors influence the rate of enzymatic reactions. These factors can be manipulated in food processing to control enzyme activity.
- Temperature: Enzyme activity generally increases with temperature until an optimum temperature is reached. Beyond this point, the enzyme can denature and lose its activity. For example, most enzymes have an optimum temperature between 30°C and 50°C, while higher temperatures denature the protein structure, leading to inactivation.
- pH: Enzymes have an optimal pH range. Changes in pH can alter the enzyme’s three-dimensional structure and affect its ability to bind to the substrate. For example, the enzyme pepsin, found in the stomach, functions optimally at a low pH (acidic), while other enzymes like amylase work best at a neutral pH.
- Substrate Concentration: As the substrate concentration increases, the rate of the enzymatic reaction increases until the enzyme becomes saturated with substrate. At this point, increasing the substrate concentration further has no effect on the reaction rate.
- Enzyme Concentration: The rate of reaction is directly proportional to the enzyme concentration, assuming sufficient substrate is available.
- Inhibitors: Certain substances can inhibit enzyme activity. These inhibitors can be competitive (competing with the substrate for the active site) or non-competitive (binding to a different site and altering the enzyme’s shape).
Examples of Enzymatic Reactions in Food Processing and Preservation
Enzymatic reactions are central to numerous food processing and preservation techniques. Understanding and controlling these reactions is key to producing safe, high-quality food products.
- Browning Reactions: The enzymatic browning of fruits and vegetables, catalyzed by enzymes like polyphenol oxidase (PPO), is a common example. This reaction leads to undesirable color changes and can be controlled by heat treatment (blanching), the addition of antioxidants (e.g., ascorbic acid), or the use of low-oxygen storage.
- Ripening of Fruits: Enzymes play a critical role in fruit ripening. For example, the enzyme pectinase breaks down pectin, softening the fruit’s texture. Starch is converted to sugar by amylase, which changes the flavor.
- Meat Tenderization: Enzymes naturally present in meat, such as cathepsins, break down muscle proteins, resulting in tenderization. This process is often accelerated during aging of meat.
- Fermentation: Enzymes are crucial in fermentation processes. For example, yeast uses enzymes like zymase to convert sugars into ethanol and carbon dioxide during beer and wine production.
- Cheese Production: Rennet, containing the enzyme chymosin, is used to coagulate milk proteins, forming curds in cheese production.
Use of Enzymes in Food Product Production
Enzymes are deliberately added or utilized in numerous food production processes to achieve specific outcomes, from altering texture to enhancing flavor.
- Cheese Production:
- Chymosin (rennet) is used to coagulate milk, forming curds.
- Lipases can be added to cheese to develop flavor through the breakdown of fats.
- Lactase can be used to reduce lactose content in cheese for lactose-intolerant consumers.
- Bread Production:
- Amylases are used to break down starch into fermentable sugars, which feed the yeast and improve the bread’s texture and crust color.
- Proteases can be used to modify gluten structure, improving dough handling and texture.
- Fruit Juice Production:
- Pectinases are used to clarify fruit juices by breaking down pectin, which causes cloudiness. This also increases juice yield.
- Amylases are used to break down starch in fruit juices, preventing cloudiness and improving clarity.
- Brewing:
- Amylases are used to break down starch in barley (or other grains) into fermentable sugars.
- Proteases are used to break down proteins, affecting foam stability and clarity.
- Production of High-Fructose Corn Syrup (HFCS):
- Glucose isomerase is used to convert glucose to fructose.
Food Colors and Pigments
Food colors and pigments are essential components of food products, significantly influencing consumer perception and acceptance. They contribute to the visual appeal of food, playing a crucial role in how we perceive its flavor and quality. Understanding the nature, stability, and application of these coloring agents is vital for food scientists and technologists.
Types of Food Pigments: Sources and Properties
Food pigments are natural or synthetic substances that impart color to food. They are classified based on their chemical structure and origin. These pigments interact with light, absorbing specific wavelengths and reflecting others, which is perceived as color.
- Carotenoids: These are a large group of tetraterpenoid pigments responsible for yellow, orange, and red colors. They are found in plants and some microorganisms.
- Source: Carrots, tomatoes, paprika, and algae.
- Properties: They are generally fat-soluble and sensitive to light, oxygen, and heat. Common examples include beta-carotene (orange), lycopene (red), and lutein (yellow). Beta-carotene is a precursor to vitamin A.
- Anthocyanins: These water-soluble pigments are responsible for red, purple, and blue colors. They are found in fruits, vegetables, and flowers.
- Source: Berries, grapes, red cabbage, and cherries.
- Properties: Their color is pH-dependent, ranging from red in acidic conditions to blue in alkaline conditions. They are sensitive to light, heat, and oxidation.
- Chlorophylls: These pigments are responsible for the green color in plants and are essential for photosynthesis.
- Source: Spinach, kale, and other green leafy vegetables.
- Properties: They are fat-soluble and sensitive to heat and acid, which can convert chlorophyll to pheophytin (olive green).
- Betalains: These water-soluble pigments are found in plants of the Caryophyllales order, such as beets.
- Source: Beets and prickly pear.
- Properties: They are relatively stable to pH changes but can be degraded by heat and light. They consist of betacyanins (red-violet) and betaxanthins (yellow-orange).
- Annatto: Derived from the seeds of the achiote tree ( Bixa orellana), annatto is a natural food coloring agent used to impart a yellow-orange hue.
- Source: Seeds of the achiote tree.
- Properties: Annatto contains carotenoid pigments, primarily bixin and norbixin. Bixin is fat-soluble, while norbixin is water-soluble. It is relatively stable to heat and light.
- Synthetic Food Colors: These are artificially produced pigments.
- Source: Synthetically produced from petroleum.
- Properties: They are generally more stable than natural colors but have raised concerns about potential health effects, leading to regulatory restrictions and consumer preferences for natural alternatives. Examples include Tartrazine (Yellow 5), Sunset Yellow FCF (Yellow 6), and Allura Red AC (Red 40).
Factors Affecting the Stability of Food Colors
The stability of food colors is crucial for maintaining the desired appearance of food products throughout their shelf life. Several factors influence the degradation of these pigments.
- Light: Exposure to light, especially ultraviolet (UV) light, can cause fading and degradation of many food colors, particularly carotenoids and anthocyanins. Packaging materials and storage conditions can mitigate this effect.
- Oxygen: Oxidation can lead to color loss, especially in carotenoids and anthocyanins. The presence of oxygen during processing and storage accelerates degradation.
- pH: The pH of the food system significantly affects the color and stability of pigments. Anthocyanins, for example, show dramatic color changes with pH variations. Chlorophyll is also sensitive to pH, undergoing transformations that alter its color.
- Heat: High temperatures can degrade pigments, leading to color loss or changes. This is particularly true for heat-sensitive pigments like anthocyanins and chlorophylls. Cooking, pasteurization, and sterilization processes can affect color stability.
- Water Activity: Water activity (aw) affects the stability of food colors. High aw can promote enzymatic and chemical reactions that degrade pigments.
- Enzymes: Some enzymes, such as peroxidases and lipoxygenases, can catalyze the degradation of pigments. Blanching or other heat treatments can inactivate these enzymes and improve color stability.
- Metal Ions: Certain metal ions can catalyze oxidation reactions and affect the color of some pigments. For example, copper and iron can catalyze the degradation of chlorophyll.
- Sulfites: The presence of sulfites can help preserve color by acting as antioxidants and reducing agents, particularly for anthocyanins.
Examples of the Use of Natural and Artificial Food Colorants
Food colorants are widely used in various food products to enhance their visual appeal. The choice between natural and artificial colorants depends on factors such as cost, stability, desired color, and regulatory considerations.
- Natural Colorants:
- Example: Yogurt colored with beetroot juice to achieve a pink hue.
- Example: Orange juice containing beta-carotene for added color.
- Example: Saffron used to color rice dishes yellow.
- Artificial Colorants:
- Example: Candies and confectionery products using Tartrazine (Yellow 5) to achieve a bright yellow color.
- Example: Soft drinks containing Allura Red AC (Red 40) for a red color.
- Example: Cake mixes using various artificial colors to create vibrant colors.
Characteristics of Common Natural Food Colorants
The following table summarizes the characteristics of common natural food colorants, including their source, color, and stability.
Colorant | Source | Color | Stability |
---|---|---|---|
Beta-Carotene | Carrots, Algae | Yellow to Orange | Good in fat-based systems; sensitive to light and oxygen. |
Anthocyanins | Berries, Grapes, Red Cabbage | Red, Purple, Blue (pH-dependent) | pH-sensitive; unstable to heat and light. |
Chlorophyll | Spinach, Kale | Green | Sensitive to heat and acid; can convert to pheophytin (olive green). |
Annatto | Achiote Seeds | Yellow-Orange | Relatively stable to heat and light. |
Food Flavors and Aromas
Food flavors and aromas are critical attributes that significantly influence consumer acceptance and enjoyment of food products. These complex sensory experiences arise from a combination of chemical compounds that interact with our taste and olfactory receptors. Understanding the nature of these compounds, their origins, and how they interact is essential for food scientists and technologists to create and maintain desirable flavor profiles in various food items.
Nature of Flavor and Aroma Compounds
Flavor perception is a multifaceted process, involving both taste and smell. Taste primarily detects the basic sensations of sweet, sour, salty, bitter, and umami, while aroma contributes significantly to the overall flavor experience. The flavor of food is determined by a combination of volatile and non-volatile compounds.
- Volatile Compounds: These are the aromatic compounds that evaporate at room temperature and reach the olfactory receptors in the nasal cavity, contributing significantly to the aroma. They are often present in low concentrations but possess high sensory impact. Examples include esters, aldehydes, ketones, and terpenes.
- Non-Volatile Compounds: These compounds contribute to the taste of food and also influence flavor perception. They include sugars, amino acids, organic acids, and salts. Their concentration and interaction with other components affect the overall taste profile.
Classes of Flavor Compounds and Their Sources
Flavor compounds originate from various sources, including raw ingredients, processing methods, and reactions within the food matrix. Identifying these sources helps in controlling and manipulating flavor development.
- Amino Acids and Peptides: These compounds, particularly glutamates, contribute to umami taste. They are found naturally in foods like tomatoes and aged cheeses, and they are also produced during protein hydrolysis.
- Sugars: Sugars, such as glucose and fructose, provide sweetness and can undergo Maillard reactions during heating, producing complex flavor compounds.
- Organic Acids: Citric acid, malic acid, and acetic acid provide sourness and influence flavor balance.
- Lipids: Lipids contribute to flavor through the release of volatile compounds during oxidation and thermal degradation.
- Sulfur Compounds: These compounds, found in onions, garlic, and cruciferous vegetables, contribute pungent and savory flavors.
- Terpenes: Terpenes, abundant in fruits and herbs, contribute fruity, floral, and herbal notes.
- Esters: Esters, produced through reactions between alcohols and acids, often provide fruity and sweet aromas.
- Aldehydes and Ketones: These compounds are often formed through oxidation and contribute to a wide range of flavors, including green, nutty, and fruity notes.
Flavor Interactions and Their Impact
Flavor compounds interact with each other and with other food components, leading to complex sensory experiences. These interactions can enhance, mask, or modify the perceived flavor.
- Synergistic Effects: Some flavor compounds enhance each other’s perceived intensity. For example, the combination of monosodium glutamate (MSG) and inosine monophosphate (IMP) creates a synergistic umami effect, enhancing the savory taste.
- Masking Effects: Certain compounds can mask or reduce the perception of other flavors. For instance, high sugar concentrations can mask bitterness.
- Suppression Effects: The presence of one flavor can reduce the perception of another. For example, the bitterness of caffeine can be suppressed by sweetness.
- Matrix Effects: The food matrix, including its physical structure and composition, can influence flavor perception. For example, fat can carry and release flavor compounds, enhancing the overall flavor experience.
- Temperature Effects: Temperature significantly influences flavor perception. Volatile compounds are more readily released at higher temperatures, enhancing aroma and flavor.
Methods for Flavor Extraction and Analysis
Flavor extraction and analysis are crucial for understanding and controlling flavor profiles in food products. Various methods are employed to isolate, identify, and quantify flavor compounds.
- Extraction Methods:
- Steam Distillation: This method involves passing steam through a food sample to extract volatile compounds. The distillate is then collected and analyzed. This is a common method for extracting essential oils.
- Solvent Extraction: This method uses organic solvents to extract flavor compounds from the food matrix. The solvent is then evaporated, leaving behind the concentrated flavor extract. Examples include Soxhlet extraction and maceration.
- Supercritical Fluid Extraction (SFE): This method uses supercritical carbon dioxide (CO2) to extract flavor compounds. SFE is often preferred because CO2 is non-toxic and leaves no residue.
- Headspace Extraction: This method involves collecting the volatile compounds from the headspace above a food sample. Techniques include static headspace and dynamic headspace (purge-and-trap).
- Analytical Methods:
- Gas Chromatography (GC): GC separates volatile flavor compounds based on their boiling points and polarity. It is a fundamental technique for flavor analysis.
- Gas Chromatography-Mass Spectrometry (GC-MS): GC-MS combines GC with mass spectrometry to identify and quantify flavor compounds. The mass spectrometer provides information on the molecular weight and fragmentation patterns of the compounds.
- High-Performance Liquid Chromatography (HPLC): HPLC is used to separate non-volatile flavor compounds, such as sugars, acids, and pigments.
- Electronic Nose: An electronic nose is a device that uses an array of sensors to detect and analyze volatile compounds, mimicking the human olfactory system.
- Sensory Evaluation: Sensory evaluation involves trained panelists evaluating the flavor characteristics of food samples using descriptive analysis, difference tests, and preference tests.
Food Additives and Preservatives
Food additives and preservatives play a crucial role in modern food processing, contributing significantly to the safety, quality, and shelf life of the foods we consume. These substances are intentionally added to food products to achieve specific technological functions, ranging from enhancing flavor and color to preventing spoilage and maintaining nutritional value. Understanding the purpose and impact of these additives is essential for both food scientists and consumers.
Role of Food Additives and Preservatives in Food Processing
Food additives and preservatives are integral components of modern food production. Their primary roles encompass several key areas, including: extending shelf life, enhancing sensory properties, improving nutritional value, and ensuring food safety. The use of these substances allows for the preservation of food over extended periods, facilitating transportation, distribution, and storage, while maintaining its quality and palatability. They also help in creating a wide variety of food products with consistent characteristics.
Types of Food Additives and Their Functions
A diverse range of food additives is employed in food processing, each serving a specific purpose. These additives are categorized based on their primary function.
- Preservatives: These additives inhibit the growth of microorganisms, such as bacteria, yeasts, and molds, thereby preventing spoilage and extending shelf life. Examples include benzoates, sorbates, and sulfites.
- Coloring Agents: These substances enhance or restore the color of food, making it more visually appealing. They can be natural (e.g., carotenoids, anthocyanins) or synthetic (e.g., tartrazine, sunset yellow).
- Flavor Enhancers: These additives improve or modify the taste and aroma of food. A common example is monosodium glutamate (MSG).
- Emulsifiers: These substances help to mix ingredients that would normally separate, such as oil and water, creating a stable emulsion. Lecithin is a common example.
- Stabilizers and Thickeners: These additives provide texture and consistency to food products, preventing separation and improving mouthfeel. Examples include gums, starches, and modified celluloses.
- Acids, Bases, and Buffering Agents: These substances control the pH of food, influencing flavor, texture, and stability. They can also affect the activity of preservatives.
- Sweeteners: These additives provide sweetness to food, either by adding calories (e.g., sugar) or without (e.g., artificial sweeteners like aspartame).
- Antioxidants: These substances prevent or slow down the oxidation of food, which can lead to rancidity, discoloration, and loss of nutrients. Examples include vitamin C (ascorbic acid) and vitamin E (tocopherol).
Use of Preservatives to Extend Shelf Life
Preservatives are crucial in extending the shelf life of various food products. Their mechanisms of action vary, but they all aim to control microbial growth and enzymatic activity.
- Pickling: This involves the use of acids (e.g., acetic acid in vinegar) and/or salt to inhibit microbial growth. This method is used to preserve vegetables like cucumbers and peppers.
- Canning: This involves heating food to high temperatures to kill microorganisms and then sealing it in airtight containers. This process is widely used for fruits, vegetables, and meats.
- Refrigeration and Freezing: While not strictly preservatives, these methods slow down microbial growth and enzymatic reactions, extending the shelf life of food.
- Addition of Chemical Preservatives: This involves the direct addition of preservatives like benzoates, sorbates, and nitrites to food products. These are commonly used in processed foods, such as soft drinks, baked goods, and cured meats.
Main Types of Preservatives
The table below summarizes the main types of preservatives, their mechanisms of action, and the foods in which they are commonly used.
Preservative Type | Mechanism of Action | Foods Commonly Used In | Examples |
---|---|---|---|
Benzoates | Inhibit the growth of yeasts and molds; effective at low pH. | Soft drinks, fruit juices, salad dressings, jams. | Sodium benzoate, potassium benzoate |
Sorbates | Inhibit the growth of molds, yeasts, and some bacteria; effective over a wider pH range than benzoates. | Cheese, baked goods, fruit products, margarine. | Potassium sorbate, calcium sorbate |
Sulfites | Inhibit microbial growth; act as antioxidants; prevent enzymatic browning. | Wines, dried fruits, processed vegetables, seafood. | Sodium sulfite, potassium metabisulfite |
Nitrites/Nitrates | Inhibit the growth of Clostridium botulinum; contribute to the characteristic color and flavor of cured meats. | Cured meats (e.g., bacon, ham, sausages). | Sodium nitrite, sodium nitrate |
Propionates | Inhibit the growth of molds and some bacteria. | Baked goods, cheese, processed meat products. | Sodium propionate, calcium propionate |
Food Processing Techniques
Food processing techniques are crucial in modern food science, extending shelf life, enhancing safety, and improving the sensory attributes of food products. These techniques utilize various methods to control microbial growth, enzymatic activity, and chemical reactions that lead to spoilage and quality degradation. Understanding the principles behind these techniques is essential for food scientists and anyone involved in food production.
Principles of Food Preservation Methods
Food preservation relies on several key principles to inhibit spoilage and maintain food quality. These methods primarily target the factors that contribute to food deterioration: microbial growth, enzymatic reactions, and chemical reactions like oxidation.
- Heating: Heat treatment, such as cooking, pasteurization, and sterilization, eliminates or reduces the number of spoilage microorganisms and inactivates enzymes. The effectiveness of heating depends on temperature, time, and the type of food. For instance, canning involves heating food in sealed containers to a high temperature to destroy all microorganisms and their spores, ensuring long-term preservation.
- Cooling: Refrigeration and freezing slow down microbial growth and enzymatic reactions by reducing the temperature. Refrigeration typically slows down spoilage processes, while freezing effectively halts them. Freezing preserves food for extended periods by converting water into ice, making it unavailable for microbial activity. The rate of freezing and the storage temperature influence the quality of the frozen product.
- Drying: Drying removes water from food, making it unavailable for microbial growth and enzymatic reactions. This can be achieved through various methods like sun-drying, air-drying, or freeze-drying. The water activity (aw) of the food is significantly reduced, inhibiting microbial proliferation. Examples include sun-dried tomatoes, air-dried fruits, and freeze-dried coffee.
- Other Methods: Other preservation techniques include the addition of preservatives (e.g., salt, sugar, acids), modified atmosphere packaging (MAP), irradiation, and fermentation. These methods work by creating unfavorable conditions for microbial growth or enzymatic activity.
Effects of Processing Techniques on Food Quality
Food processing techniques can significantly affect the quality of food products, influencing their nutritional value, sensory characteristics (appearance, flavor, texture), and safety. It is crucial to consider these effects when selecting and applying processing methods.
- Nutritional Changes: Processing can impact the nutritional content of food. Heat treatment can lead to the loss of heat-sensitive vitamins like vitamin C and thiamin. However, some processing methods, like cooking, can also improve the bioavailability of certain nutrients. For example, cooking tomatoes increases the bioavailability of lycopene.
- Sensory Changes: Processing techniques can alter the sensory attributes of food. Heating can affect the color, flavor, and texture of food. For instance, caramelization and Maillard reactions occur during heating, producing desirable flavors and colors in foods like bread and roasted meats. Freezing can affect the texture of food, sometimes leading to ice crystal formation that impacts the texture.
- Safety Considerations: Processing plays a vital role in ensuring food safety. Heat treatment effectively eliminates harmful microorganisms. Proper processing also inactivates enzymes that can cause spoilage and reduces the risk of foodborne illnesses. The selection of appropriate processing parameters is crucial to guarantee the safety of the final product.
- Shelf Life: Processing techniques significantly extend the shelf life of food products by controlling microbial growth, enzymatic reactions, and chemical changes. Canning, for example, allows for the long-term storage of food at room temperature. The shelf life extension depends on the processing method and the type of food.
Examples of Modern Food Processing Technologies
Modern food processing technologies have evolved to improve efficiency, enhance food quality, and ensure food safety. These technologies often incorporate automation, precise control, and innovative methods.
- High-Pressure Processing (HPP): HPP uses ultra-high pressure to inactivate microorganisms and enzymes without the use of heat. This technology helps retain the fresh-like characteristics of food products. HPP is used in the production of fruit juices, ready-to-eat meals, and deli meats.
- Pulsed Electric Field (PEF): PEF technology uses short pulses of high-voltage electricity to disrupt cell membranes of microorganisms, leading to their inactivation. This is a non-thermal processing method used to preserve liquid foods like juices and milk, reducing the need for heat treatment.
- Microwave Processing: Microwave heating is used for various applications, including thawing, cooking, and pasteurization. It offers rapid and uniform heating, which can help preserve the nutritional value and sensory quality of food. Microwave technology is widely used in the food industry for ready-to-eat meals and snacks.
- Membrane Filtration: Membrane filtration technologies, such as microfiltration, ultrafiltration, and reverse osmosis, are used to separate components of food products based on their size and properties. These methods are used for clarifying juices, concentrating milk, and removing bacteria from liquids.
- Extrusion: Extrusion is a continuous process where food materials are forced through a die under high pressure and temperature. This technology is used to create a variety of food products, including breakfast cereals, pasta, and snacks.
Pasteurization: Principles, Procedures, and Effects
Pasteurization is a heat treatment process designed to kill pathogenic microorganisms in food while minimizing changes in the food’s quality. It is named after Louis Pasteur, who first used it to prevent the spoilage of wine. Pasteurization is widely used in the dairy, juice, and egg industries.
- Principles: Pasteurization aims to reduce the number of viable microorganisms to a level that the food is safe for consumption and extends its shelf life. The process involves heating the food to a specific temperature for a specific time, which is determined by the type of food and the target microorganisms. The primary goal is to eliminate pathogenic bacteria like
-Salmonella*,
-Listeria*, and
-E.coli* while minimizing the impact on the food’s sensory and nutritional properties.
- Procedures: The pasteurization process varies depending on the food product.
- Batch Pasteurization: This method involves heating the food in a tank or vat to a specific temperature for a specific time. For example, milk may be heated to 63°C (145°F) for 30 minutes.
- High-Temperature Short-Time (HTST) Pasteurization: This is the most common method used in the dairy industry. The food is heated to a higher temperature for a shorter time. For example, milk is typically heated to 72°C (161°F) for 15 seconds.
- Ultra-High Temperature (UHT) Pasteurization: This process involves heating the food to a very high temperature (e.g., 135°C or 275°F) for a very short time (e.g., 2-5 seconds). UHT pasteurization is used to produce shelf-stable products like milk and juice.
- Effects on Different Food Products: The effects of pasteurization vary depending on the food product.
- Milk: Pasteurization eliminates pathogenic bacteria in milk, making it safe for consumption. HTST pasteurization minimally affects the flavor and nutritional value of milk. UHT pasteurization can result in slight changes in flavor and a longer shelf life.
- Juices: Pasteurization inactivates spoilage organisms and enzymes in juices, extending their shelf life. It also reduces the risk of foodborne illnesses. Pasteurization may slightly affect the flavor and vitamin content of juices.
- Eggs: Pasteurization of eggs is used to eliminate
-Salmonella*, which is a common concern. The process is carefully controlled to avoid cooking the eggs.
Food Packaging and Storage
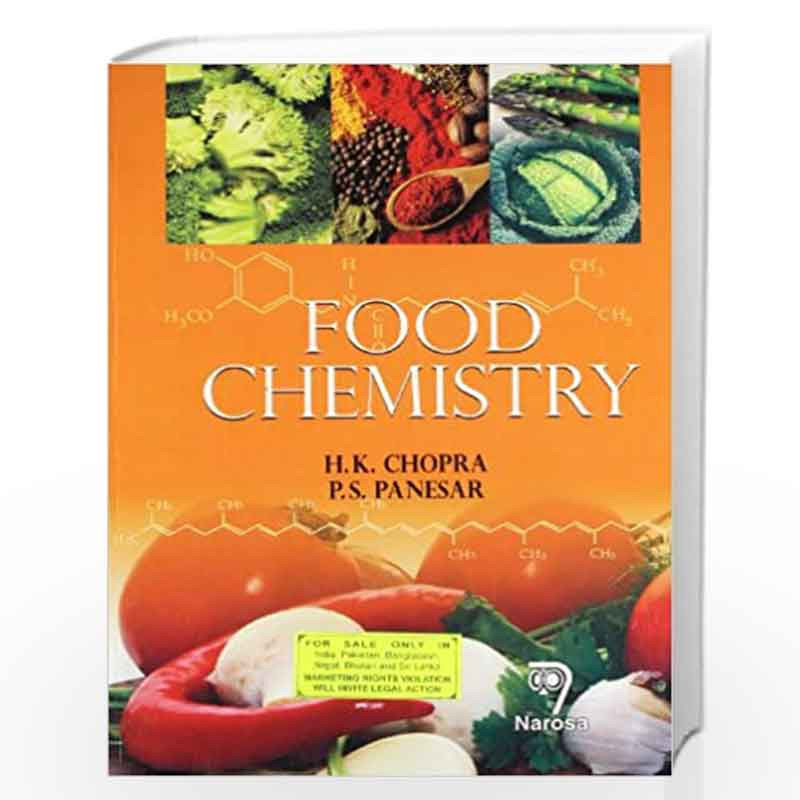
Food packaging and storage are critical components of the food supply chain, playing a vital role in maintaining food quality, safety, and extending shelf life. Effective packaging and storage strategies protect food products from physical, chemical, and biological degradation, ensuring that consumers receive safe and palatable food. These practices are crucial in minimizing food waste and facilitating the global distribution of food products.
Role of Food Packaging in Preserving Food Quality and Safety
Food packaging acts as a barrier, safeguarding food from various external factors that can compromise its quality and safety. This protection encompasses several key aspects:
- Physical Protection: Packaging shields food from physical damage during handling, transportation, and storage. This includes preventing crushing, bruising, and punctures, which can lead to spoilage and contamination.
- Protection from Environmental Factors: Packaging prevents or minimizes the exposure of food to environmental elements that can cause degradation. These include:
- Oxygen: Oxygen can cause oxidative rancidity in fats, leading to off-flavors and spoilage. Packaging that limits oxygen permeability helps to preserve food quality.
- Moisture: Moisture can affect the texture, appearance, and microbial stability of food. Packaging controls moisture transfer, preventing food from becoming soggy or dry.
- Light: Light, especially ultraviolet (UV) light, can degrade food components, leading to color changes, flavor loss, and nutrient degradation. Opaque or UV-protective packaging helps to mitigate these effects.
- Temperature: Packaging can help maintain the desired temperature of food products, whether it’s refrigeration, freezing, or ambient storage. This is especially important for temperature-sensitive foods like dairy products and frozen foods.
- Prevention of Contamination: Packaging serves as a barrier against contaminants, including microorganisms, insects, and other foreign materials. This is crucial for food safety and preventing foodborne illnesses.
- Information and Communication: Packaging provides essential information to consumers, including product ingredients, nutritional information, expiration dates, and handling instructions. It also facilitates branding and marketing efforts.
Different Types of Food Packaging Materials
A wide range of materials is used for food packaging, each offering specific properties and advantages. The selection of the appropriate material depends on the food product’s characteristics, desired shelf life, and the intended distribution and storage conditions.
- Plastics: Plastics are one of the most widely used packaging materials due to their versatility, cost-effectiveness, and barrier properties.
- Polyethylene (PE): Commonly used for flexible packaging, such as films, bags, and pouches. It offers good moisture barrier properties and is relatively inexpensive. Examples include milk jugs, plastic wraps, and squeeze bottles.
- Polypropylene (PP): Used for rigid and flexible packaging, PP is heat-resistant and suitable for hot-fill applications. It is commonly used for containers for yogurt, margarine, and microwaveable food.
- Polyethylene terephthalate (PET): A clear, strong, and lightweight plastic, PET is often used for bottles, jars, and trays. It provides good barrier properties against oxygen and carbon dioxide and is commonly used for soft drink bottles and salad dressing containers.
- Polystyrene (PS): Available in both rigid and foam forms, PS is used for packaging food trays, cups, and containers. Expanded polystyrene (EPS) foam is often used for insulation and cushioning.
- Glass: Glass is an inert material that provides excellent barrier properties against oxygen, moisture, and other contaminants. It is also transparent, allowing consumers to view the product.
- Glass jars and bottles are commonly used for preserving food products like jams, pickles, and sauces.
- Glass is recyclable and can be reused, making it an environmentally friendly option.
- Metals: Metals, particularly steel and aluminum, are used for packaging food products that require a high degree of protection and long shelf life.
- Steel Cans: Steel cans are strong and provide excellent barrier properties. They are commonly used for canned fruits, vegetables, and meats. The interior of the can is often coated with a protective layer to prevent corrosion and interaction with the food.
- Aluminum Cans and Foil: Aluminum is lightweight, corrosion-resistant, and provides good barrier properties. It is used for beverage cans, foil wraps, and flexible packaging.
- Paper and Paperboard: Paper and paperboard are renewable and biodegradable materials. They are often used for packaging dry foods and providing structural support.
- Paperboard Cartons: Used for packaging cereals, crackers, and other dry goods. They can be coated with other materials to enhance barrier properties.
- Corrugated Cardboard: Used for shipping boxes and provides cushioning to protect products during transportation.
- Composites: Composite materials combine different materials to create packaging with enhanced properties.
- Tetra Pak: A common example of composite packaging, Tetra Pak cartons combine paperboard, polyethylene, and aluminum foil to provide excellent barrier properties for liquid products like milk and juice.
Appropriate Packaging and Storage Methods for Different Food Products
The selection of packaging and storage methods varies greatly depending on the type of food product. Different food products require specific packaging and storage strategies to maintain their quality, safety, and shelf life.
- Fresh Produce:
- Packaging: Perforated plastic bags, clamshell containers, and trays with film wrapping are commonly used. The packaging should allow for some air circulation to prevent moisture buildup and spoilage.
- Storage: Refrigeration at appropriate temperatures (e.g., 0-5°C for many fruits and vegetables) is essential. Controlled atmosphere storage (CAS) and modified atmosphere packaging (MAP) can extend shelf life by controlling the levels of oxygen, carbon dioxide, and ethylene gas.
- Meat and Poultry:
- Packaging: Vacuum packaging, modified atmosphere packaging (MAP), and shrink wrapping are used to extend shelf life and maintain product quality. Vacuum packaging removes oxygen, inhibiting the growth of spoilage organisms. MAP involves altering the gas composition within the package to slow down spoilage.
- Storage: Refrigeration at temperatures below 4°C is crucial. Frozen storage at -18°C or lower is recommended for long-term preservation.
- Dairy Products:
- Packaging: Milk is often packaged in plastic jugs or cartons, which provide a barrier against light and oxygen. Yogurt and cheese are packaged in various containers, including plastic tubs, foil-lined cartons, and plastic film.
- Storage: Refrigeration at temperatures below 4°C is necessary to prevent bacterial growth and spoilage.
- Baked Goods:
- Packaging: Bread and pastries are often packaged in plastic bags or paperboard boxes. The packaging should provide a barrier against moisture loss and maintain product freshness. Modified atmosphere packaging (MAP) can also be used to extend shelf life.
- Storage: Proper storage depends on the type of baked good. Bread is often stored at room temperature, while pastries may require refrigeration. Freezing is a common method for extending the shelf life of baked goods.
- Dry Foods (Cereals, Snacks, etc.):
- Packaging: Packaging should provide a barrier against moisture and oxygen to maintain crispness and prevent spoilage. Flexible packaging (e.g., plastic films, pouches) and paperboard boxes are commonly used. The packaging may include a re-sealable feature.
- Storage: Store in a cool, dry place. Once opened, reseal the packaging or transfer the food to an airtight container.
- Canned Foods:
- Packaging: Canned foods are packaged in hermetically sealed metal cans or glass jars. The canning process involves heat treatment to sterilize the food and destroy spoilage organisms.
- Storage: Store in a cool, dry place. Canned foods have a long shelf life when stored properly. Avoid storing cans that are dented, bulging, or leaking.
Factors to Consider When Choosing Food Packaging Materials
Selecting the appropriate packaging material is a complex process, involving a careful consideration of various factors. These factors influence the performance, cost-effectiveness, and environmental impact of the packaging.
- Food Product Characteristics: The properties of the food product, such as its composition, pH, water activity, and sensitivity to light and oxygen, significantly influence the choice of packaging material. For instance, oxygen-sensitive foods require packaging with high barrier properties.
- Barrier Properties: The packaging material’s ability to prevent the passage of gases (oxygen, carbon dioxide), water vapor, and other substances is crucial for maintaining food quality and extending shelf life. The desired barrier properties vary depending on the food product and its storage conditions.
- Mechanical Properties: The packaging material’s strength, flexibility, and resistance to puncture, tearing, and crushing are important for protecting the food product during handling, transportation, and storage.
- Cost: The cost of the packaging material and the overall packaging system is a significant consideration. Packaging costs must be balanced against the desired performance and shelf life of the food product.
- Processing and Filling Requirements: The packaging material must be compatible with the food processing and filling equipment used. This includes factors like heat resistance, sealability, and machinability.
- Shelf Life Requirements: The desired shelf life of the food product dictates the level of protection and barrier properties needed from the packaging. Longer shelf life typically requires packaging with higher barrier properties and more robust sealing.
- Sustainability and Environmental Impact: Increasingly, the environmental impact of packaging is a key consideration. This includes the use of renewable resources, recyclability, and the potential for composting. Sustainable packaging solutions are becoming increasingly important.
- Regulatory Compliance: Food packaging materials must comply with relevant food safety regulations, such as those set by the Food and Drug Administration (FDA) in the United States or the European Food Safety Authority (EFSA) in Europe. These regulations address the safety of packaging materials and their potential migration of substances into the food.
- Aesthetics and Marketing: The appearance of the packaging is important for attracting consumers and communicating brand identity. This includes factors like printability, clarity, and the overall design of the packaging.
- Distribution and Storage Conditions: The intended distribution and storage conditions, including temperature, humidity, and transportation methods, influence the choice of packaging material. For example, frozen foods require packaging that can withstand low temperatures and prevent freezer burn.
Food Safety and Quality Control
Ensuring the safety and quality of food products is paramount in the food industry. This involves a multifaceted approach encompassing various control measures, from the raw material stage to the final consumer. Implementing robust food safety and quality control systems protects public health, maintains consumer confidence, and ensures compliance with regulatory standards. These systems also contribute to reducing food waste and economic losses.
Principles of Food Safety and Quality Control
Food safety and quality control are built upon several core principles. These principles guide the development and implementation of effective food safety and quality management systems.
- Prevention over Reaction: The primary focus is on preventing hazards rather than reacting to them. This proactive approach involves identifying potential risks and implementing control measures before they compromise food safety or quality.
- Hazard Analysis and Risk Assessment: This involves systematically identifying potential hazards (biological, chemical, and physical) that may occur in the food production process. A risk assessment is then conducted to evaluate the likelihood and severity of each hazard.
- Critical Control Points (CCPs): CCPs are specific points in the food production process where control is essential to prevent or eliminate a food safety hazard or reduce it to an acceptable level.
- Monitoring and Verification: Regular monitoring of CCPs is crucial to ensure that control measures are effective. Verification activities, such as audits and testing, confirm that the system is functioning as intended.
- Corrective Actions: Established procedures for taking corrective actions are essential when monitoring reveals that a CCP is not under control. This includes identifying the root cause of the problem and implementing measures to prevent recurrence.
- Documentation and Record Keeping: Detailed documentation of all aspects of the food safety and quality control system is critical. This includes procedures, monitoring records, corrective actions, and verification results. Proper record-keeping facilitates traceability and helps in investigations if problems arise.
- Continuous Improvement: Food safety and quality control systems should be continuously evaluated and improved based on new scientific information, technological advancements, and feedback from consumers and regulatory agencies.
Common Foodborne Hazards and Their Sources
Foodborne hazards can be broadly classified into biological, chemical, and physical hazards. Understanding these hazards and their sources is essential for implementing effective control measures.
- Biological Hazards: These include bacteria, viruses, parasites, and fungi.
- Bacteria: Salmonella, Escherichia coli ( E. coli), Listeria monocytogenes, and Clostridium botulinum are examples of pathogenic bacteria. Sources include raw meat, poultry, eggs, and contaminated water. For instance, Salmonella is frequently associated with poultry products, causing salmonellosis.
- Viruses: Norovirus and Hepatitis A are common foodborne viruses. Sources include contaminated food handlers and contaminated water. Norovirus outbreaks are often linked to the consumption of raw or undercooked shellfish.
- Parasites: Giardia, Cryptosporidium, and Trichinella spiralis are examples of foodborne parasites. Sources include contaminated water and undercooked meat. Trichinella spiralis can be found in undercooked pork.
- Fungi: Mycotoxins produced by fungi, such as aflatoxins, are a concern. Sources include moldy grains and nuts. Aflatoxins are particularly associated with peanuts and corn.
- Chemical Hazards: These include toxins, allergens, and contaminants.
- Toxins: Naturally occurring toxins (e.g., mycotoxins, scombroid poisoning from histamine in fish) and those from chemical contamination (e.g., pesticides, heavy metals). Sources include contaminated soil, water, and improper food handling.
- Allergens: Major food allergens (e.g., milk, eggs, peanuts, tree nuts, soy, wheat, fish, and shellfish). Sources include ingredients and cross-contamination during processing. Proper labeling and allergen management procedures are crucial to prevent allergic reactions.
- Contaminants: Chemicals, cleaning agents, and packaging materials. Sources include improper storage, cross-contamination, and migration from packaging materials.
- Physical Hazards: These include foreign objects that can cause injury.
- Examples: Glass shards, metal fragments, plastic pieces, stones, and bones. Sources include equipment malfunction, poor handling practices, and inadequate quality control.
Quality Control Measures in Food Production
A variety of quality control measures are employed throughout the food production process to ensure product safety and quality. These measures are implemented at different stages, from raw material procurement to packaging and distribution.
- Supplier Approval Programs: Evaluating and approving suppliers based on their ability to meet food safety and quality standards. This involves audits, inspections, and the review of supplier documentation.
- Raw Material Inspection: Inspecting raw materials upon arrival to ensure they meet specifications, are free from contamination, and are properly handled. This may involve visual inspection, laboratory testing, and sensory evaluation.
- Process Control: Implementing control measures at each stage of the production process to minimize the risk of hazards. This includes monitoring critical parameters, such as temperature, time, and pH.
- Equipment Maintenance: Regularly maintaining and calibrating equipment to ensure it functions correctly and does not contribute to contamination. This includes cleaning and sanitation procedures.
- Personnel Hygiene: Training and enforcing strict hygiene practices for all food handlers. This includes handwashing, proper attire, and preventing cross-contamination.
- Sanitation Programs: Implementing and monitoring effective cleaning and sanitation procedures to prevent the growth of microorganisms and eliminate contamination.
- Packaging and Storage: Using appropriate packaging materials and storage conditions to protect the product from contamination and maintain its quality.
- Product Testing: Conducting laboratory testing to verify that the product meets safety and quality standards. This includes microbiological analysis, chemical analysis, and sensory evaluation.
- Traceability Systems: Implementing systems to track the movement of food products throughout the supply chain. This allows for rapid identification and removal of contaminated products in case of a recall.
HACCP System: Principles, Steps, and Benefits
HACCP (Hazard Analysis and Critical Control Points) is a systematic, science-based approach to food safety that identifies, evaluates, and controls hazards that are significant for food safety. It is a preventative system that focuses on controlling hazards at specific points in the food production process.
Principles of HACCP
The HACCP system is based on seven key principles:
- Conduct a hazard analysis. Identify potential hazards (biological, chemical, or physical) that may be reasonably expected to occur at each step in the food production process.
- Determine the Critical Control Points (CCPs). Identify the points in the process where control is essential to prevent or eliminate a food safety hazard or reduce it to an acceptable level.
- Establish critical limits. Set specific, measurable criteria for each CCP that must be met to ensure the hazard is under control.
- Establish a monitoring system. Develop procedures to monitor the CCPs to ensure that the critical limits are met.
- Establish corrective actions. Define actions to be taken if monitoring indicates that a critical limit is not met.
- Establish verification procedures. Implement procedures to verify that the HACCP system is working effectively.
- Establish record-keeping and documentation procedures. Maintain records of all aspects of the HACCP system, including hazard analysis, CCPs, critical limits, monitoring results, corrective actions, and verification activities.
Steps in Developing a HACCP Plan
Developing a HACCP plan involves several steps:
- Assemble the HACCP team. Form a multidisciplinary team with expertise in food science, food processing, and microbiology.
- Describe the product. Provide a detailed description of the food product, including its ingredients, processing method, packaging, storage, and intended use.
- Identify the intended use and consumers of the food. Determine the target consumer group and their potential vulnerability to foodborne illness.
- Construct a flow diagram. Create a flow diagram that Artikels all steps in the food production process.
- Verify the flow diagram on-site. Confirm the accuracy of the flow diagram by observing the actual food production process.
- Conduct a hazard analysis (Principle 1). Identify potential hazards associated with each step in the process and assess the severity and likelihood of their occurrence.
- Determine the Critical Control Points (CCPs) (Principle 2). Based on the hazard analysis, identify the CCPs where control is essential.
- Establish critical limits for each CCP (Principle 3). Set specific, measurable criteria for each CCP that must be met to ensure the hazard is under control.
- Establish a monitoring system for each CCP (Principle 4). Develop procedures to monitor the CCPs to ensure that the critical limits are met. This includes specifying what will be monitored, how it will be monitored, the frequency of monitoring, and who will be responsible for monitoring.
- Establish corrective actions (Principle 5). Define the actions to be taken if monitoring indicates that a critical limit is not met. This includes identifying the root cause of the problem, correcting the problem, and preventing its recurrence.
- Establish verification procedures (Principle 6). Implement procedures to verify that the HACCP system is working effectively. This includes periodic audits, review of records, and testing.
- Establish record-keeping and documentation procedures (Principle 7). Maintain detailed records of all aspects of the HACCP system, including hazard analysis, CCPs, critical limits, monitoring results, corrective actions, and verification activities.
Benefits of Implementing HACCP
The HACCP system offers several benefits to food producers, consumers, and regulatory agencies:
- Enhanced Food Safety: HACCP helps to prevent foodborne illnesses by focusing on the control of hazards at critical points in the production process.
- Reduced Risk of Foodborne Outbreaks: By identifying and controlling hazards, HACCP minimizes the likelihood of foodborne outbreaks.
- Improved Product Quality: HACCP can also contribute to improved product quality by controlling factors that affect product characteristics.
- Increased Consumer Confidence: Implementing a HACCP system demonstrates a commitment to food safety, which increases consumer confidence in the product.
- Improved Efficiency: HACCP can help to streamline production processes and reduce waste.
- Compliance with Regulatory Requirements: HACCP is recognized and often required by regulatory agencies worldwide.
- Cost Savings: By preventing food safety problems, HACCP can help to reduce costs associated with recalls, legal liabilities, and loss of consumer confidence.
Nutritional Aspects of Food: Food Chemistry Textbook
Understanding the nutritional aspects of food is fundamental to comprehending how what we eat impacts our health and well-being. This chapter delves into the composition of food, exploring the essential nutrients that fuel our bodies and the crucial roles they play in maintaining optimal health. It also provides insights into dietary guidelines and recommendations designed to promote a balanced and healthful diet.
Nutritional Composition of Foods
Foods are complex mixtures of various substances, but from a nutritional perspective, they are primarily composed of macronutrients and micronutrients. The proportions of these components vary significantly depending on the food source.
- Macronutrients: These are nutrients required in relatively large amounts to provide energy and support bodily functions. They include:
- Carbohydrates: The primary source of energy for the body. They are found in grains, fruits, vegetables, and legumes.
Example: A slice of bread provides approximately 15 grams of carbohydrates.
- Proteins: Essential for building and repairing tissues, as well as producing enzymes and hormones. They are found in meat, poultry, fish, beans, and dairy products.
Example: A 3-ounce serving of cooked chicken breast contains about 26 grams of protein.
- Lipids (Fats): Provide energy, insulate organs, and aid in the absorption of fat-soluble vitamins. They are found in oils, nuts, seeds, and animal products.
Example: One tablespoon of olive oil contains approximately 14 grams of fat.
- Carbohydrates: The primary source of energy for the body. They are found in grains, fruits, vegetables, and legumes.
- Micronutrients: These nutrients are required in smaller amounts but are just as vital for maintaining health. They encompass:
- Vitamins: Organic compounds that regulate various metabolic processes. They are classified as either water-soluble (e.g., Vitamin C, B vitamins) or fat-soluble (e.g., Vitamins A, D, E, K).
Example: A medium orange provides a significant amount of Vitamin C.
- Minerals: Inorganic substances that play roles in bone health, fluid balance, and nerve function. They include calcium, iron, sodium, and potassium.
Example: Dairy products are a good source of calcium.
- Vitamins: Organic compounds that regulate various metabolic processes. They are classified as either water-soluble (e.g., Vitamin C, B vitamins) or fat-soluble (e.g., Vitamins A, D, E, K).
- Water: Though not a macronutrient or micronutrient in the strictest sense, water is essential for all bodily functions. It makes up a significant portion of our body weight and is crucial for hydration and nutrient transport.
Example: Water constitutes approximately 55% to 78% of a human’s body weight.
Role of Nutrients in Human Health
Each nutrient plays a specific and often multifaceted role in maintaining human health. The absence or insufficiency of any essential nutrient can lead to various health problems.
- Carbohydrates: Primarily provide energy for the body, particularly for the brain and nervous system. Excess carbohydrate intake can lead to weight gain. Insufficient carbohydrate intake can lead to fatigue and the breakdown of muscle tissue for energy.
Example: Glucose, a simple carbohydrate, is the brain’s preferred energy source.
- Proteins: Crucial for building and repairing tissues, producing enzymes and hormones, and supporting the immune system. Protein deficiency can lead to muscle wasting, impaired immune function, and stunted growth in children.
Example: Enzymes are proteins that catalyze biochemical reactions.
- Lipids (Fats): Provide energy, insulate organs, and aid in the absorption of fat-soluble vitamins. They also play a role in hormone production. Excessive intake of saturated and trans fats can increase the risk of heart disease.
Example: Cholesterol, a type of lipid, is essential for cell membrane structure and hormone synthesis.
- Vitamins: Act as coenzymes, assisting in various metabolic processes. Vitamin deficiencies can lead to specific diseases, such as scurvy (Vitamin C deficiency) and rickets (Vitamin D deficiency).
Example: Vitamin D is essential for calcium absorption and bone health.
- Minerals: Involved in bone health, fluid balance, nerve function, and various enzymatic reactions. Deficiencies in minerals like iron and calcium can lead to anemia and osteoporosis, respectively.
Example: Calcium is a key component of bones and teeth.
- Water: Essential for hydration, nutrient transport, temperature regulation, and waste removal. Dehydration can lead to fatigue, headaches, and impaired cognitive function.
Example: Water is the primary component of blood, which transports nutrients and oxygen throughout the body.
Dietary Recommendations and Guidelines
Dietary recommendations and guidelines provide a framework for making informed food choices to promote health and prevent chronic diseases. These guidelines are typically developed by governmental or professional organizations and are based on scientific evidence.
- Dietary Guidelines for Americans: These guidelines, updated periodically, provide evidence-based advice on food and beverage choices to promote health, prevent chronic disease, and help people of all ages maintain a healthy weight.
Example: The guidelines recommend consuming a variety of nutrient-dense foods from all food groups.
- MyPlate: A visual representation of the Dietary Guidelines for Americans, MyPlate illustrates the proportions of each food group that should make up a healthy diet.
Description: MyPlate is divided into five food groups: fruits, vegetables, grains, protein foods, and dairy. It emphasizes the importance of portion control and a balanced diet.
- World Health Organization (WHO) Recommendations: The WHO provides global recommendations on healthy diets, including recommendations for reducing the intake of free sugars, saturated fats, and trans-fats, and increasing the intake of fruits, vegetables, and fiber.
Example: The WHO recommends that adults and children reduce their daily intake of free sugars to less than 10% of their total energy intake.
Key Nutrients and Their Functions
The following table summarizes the key nutrients and their primary functions in the body.
Nutrient | Main Function | Sources | Deficiency Symptoms |
---|---|---|---|
Carbohydrates | Primary energy source | Grains, fruits, vegetables, legumes | Fatigue, weakness |
Proteins | Building and repairing tissues, enzyme and hormone production | Meat, poultry, fish, beans, dairy products | Muscle wasting, impaired immune function |
Lipids (Fats) | Energy storage, insulation, absorption of fat-soluble vitamins | Oils, nuts, seeds, animal products | Fatigue, skin problems |
Vitamin C | Antioxidant, immune function, collagen synthesis | Citrus fruits, berries, peppers | Scurvy, impaired wound healing |
Vitamin D | Calcium absorption, bone health | Fatty fish, fortified foods, sunlight | Rickets, osteoporosis |
Calcium | Bone and teeth health, nerve function | Dairy products, leafy green vegetables | Osteoporosis, muscle cramps |
Iron | Oxygen transport | Red meat, beans, spinach | Anemia, fatigue |
Advanced Topics in Food Chemistry
The field of food chemistry is dynamic, constantly evolving to meet the challenges of a growing global population, changing consumer preferences, and the need for sustainable food production practices. This section delves into some of the most promising areas of current research, exploring the challenges and opportunities that lie ahead. It highlights innovative applications of food chemistry that are shaping the future of the food industry.
Emerging Trends in Food Chemistry Research
Food chemistry research is currently experiencing significant shifts, driven by technological advancements and consumer demand for healthier, more sustainable food options. Several key trends are prominent.
- Personalized Nutrition: This involves tailoring dietary recommendations based on individual genetic makeup, gut microbiome composition, and lifestyle factors. Food chemists are developing methods to analyze food components and their interactions with the human body at a personalized level. This can involve identifying specific biomarkers to determine how different foods impact individuals, with the goal of creating diets optimized for specific health outcomes.
- Plant-Based Foods and Alternative Proteins: The demand for plant-based alternatives to meat and dairy products is increasing. Research focuses on improving the texture, flavor, and nutritional profile of these products, including the use of advanced techniques like extrusion, fermentation, and enzymatic modification. The development of novel protein sources, such as insect-based proteins and cultivated meat, is also a rapidly growing area.
- Food Nanotechnology: Nanotechnology is being applied to food systems for various purposes, including enhanced delivery of nutrients, improved food packaging, and detection of foodborne pathogens. This involves the use of nanomaterials to encapsulate bioactive compounds, improve the shelf life of foods, and create sensors for food safety monitoring.
- Sustainable Food Systems: Food chemistry is contributing to the development of more sustainable food production practices. This includes research on reducing food waste, utilizing byproducts from food processing, and developing eco-friendly packaging materials. The focus is on minimizing the environmental impact of food production and ensuring food security.
- Computational Food Chemistry: Computational modeling and simulation are increasingly used to predict food properties, optimize food processing parameters, and understand complex food systems. This approach allows researchers to accelerate the development of new food products and processes by simulating experiments before conducting them in the laboratory.
Challenges and Opportunities in Food Chemistry
The field of food chemistry faces several significant challenges, but these also present opportunities for innovation and growth. Addressing these challenges is crucial for ensuring a safe, sustainable, and nutritious food supply.
- Food Security: Feeding a growing global population requires innovative solutions to increase food production while minimizing environmental impact. This involves developing drought-resistant crops, improving food storage and preservation techniques, and reducing food waste.
- Food Safety: Ensuring the safety of the food supply is a constant challenge, with the need to detect and control foodborne pathogens, allergens, and contaminants. Advanced analytical techniques and improved food processing methods are critical.
- Consumer Demand and Preferences: Consumers are increasingly demanding healthier, more convenient, and sustainable food options. This requires food chemists to develop products that meet these demands while maintaining desirable sensory properties and shelf life.
- Technological Advancements: The rapid pace of technological advancements, such as gene editing and artificial intelligence, offers both opportunities and challenges. It’s essential to leverage these technologies responsibly and ethically to improve food production and processing.
- Regulatory Frameworks: The food industry operates within complex regulatory frameworks. Navigating these regulations and ensuring compliance is crucial for bringing new products to market and maintaining consumer trust.
Innovative Applications of Food Chemistry in the Food Industry
Food chemistry plays a crucial role in developing innovative products and processes that improve food quality, safety, and sustainability. Several examples illustrate its impact.
- Encapsulation of Bioactive Compounds: Food chemists are developing encapsulation techniques to protect and deliver bioactive compounds, such as vitamins, antioxidants, and probiotics. These techniques can improve the stability and bioavailability of these compounds, leading to enhanced health benefits. For example, microencapsulation of omega-3 fatty acids in food products can prevent oxidation and improve their shelf life, while delivering these beneficial nutrients.
- Modified Atmosphere Packaging (MAP): MAP involves modifying the gas composition within food packaging to extend shelf life and maintain food quality. This technology is widely used for fresh produce, meat, and processed foods, preserving freshness and reducing the need for preservatives. The precise control of oxygen, carbon dioxide, and nitrogen within the package slows down spoilage reactions and enzymatic activity.
- Development of Novel Food Ingredients: Food chemists are constantly exploring new ingredients to improve the functionality, nutritional value, and sensory properties of food products. This includes the use of alternative proteins, prebiotics, and probiotics. For instance, the development of pea protein isolates for use in plant-based meat alternatives, providing the desired texture and protein content.
- Enzyme Technology in Food Processing: Enzymes are used in various food processing applications to improve efficiency, reduce waste, and enhance product quality. Enzymes can be used to modify starch, hydrolyze proteins, and clarify juices. For example, using pectinases to clarify fruit juices, enhancing their clarity and visual appeal.
- Food Waste Reduction: Food chemists are developing innovative approaches to reduce food waste, such as upcycling food processing byproducts into valuable ingredients. This can involve extracting bioactive compounds from fruit peels or using whey protein from cheese production in new food products.
The Impact of Food Chemistry on Sustainability and Food Security
Food chemistry plays a critical role in addressing global challenges related to sustainability and food security. Research and innovation in this field are instrumental in developing strategies to minimize environmental impact, improve resource utilization, and ensure access to safe and nutritious food for all.
One key area is the reduction of food waste. Food chemists are developing technologies to extend the shelf life of perishable foods, improve storage techniques, and convert food waste into valuable resources, such as animal feed or biofuels. The development of biodegradable packaging and sustainable processing methods also contributes to reducing the environmental footprint of the food industry.
In the realm of food security, food chemistry is crucial for enhancing crop yields and nutritional value. Researchers are working on improving the bioavailability of essential nutrients in staple foods, developing fortified foods, and creating drought-resistant crops. These efforts are vital in combating malnutrition and ensuring a stable food supply for vulnerable populations.
Furthermore, food chemistry contributes to the development of sustainable agricultural practices. This includes the use of biofertilizers, the development of pest-resistant crops, and the optimization of food processing techniques to minimize water and energy consumption. By promoting environmentally friendly practices, food chemistry is helping to create a more resilient and sustainable food system.
Final Summary
In conclusion, this food chemistry textbook provides a comprehensive overview of the science that underpins our daily meals. By exploring the complexities of food composition, processing, and preservation, we gain a deeper appreciation for the science that makes our food safe, nutritious, and enjoyable. This exploration of the chemical intricacies of food ultimately empowers us to make informed choices, fostering a healthier relationship with the food we consume.
The knowledge gained from this book is a valuable asset for anyone seeking a deeper understanding of the culinary world and its impact on our lives.