Food the delicious science, at its core, is a fascinating exploration of how everyday ingredients transform into the meals we savor. This journey delves into the intricate interplay of chemistry, physics, and biology, revealing the scientific principles that underpin every delicious bite. From the browning of a perfectly seared steak to the fluffy rise of a freshly baked loaf of bread, the science of food is constantly at work, shaping our culinary experiences in ways we might not even realize.
This article will explore various scientific disciplines that intersect with food. We’ll uncover the chemical reactions that create complex flavors, the physics that dictate cooking techniques, and the biological processes that influence our perception of taste and texture. Prepare to discover how seemingly simple actions in the kitchen are actually governed by intricate scientific principles, transforming the way you view and appreciate your next meal.
Unveiling the Delicious Science of Food
The science of food, a fascinating and complex field, explores the transformation of raw ingredients into the meals we savor. It’s a multidisciplinary area, applying scientific principles to understand how food is produced, processed, prepared, and consumed. This knowledge not only enhances our culinary experiences but also ensures food safety, improves nutritional value, and develops innovative food products.The everyday act of cooking is a laboratory of scientific principles.
From the simple act of frying an egg to the complex process of baking a cake, the science of food is constantly at play. Consider the Maillard reaction, responsible for the browning and flavor development in seared meats and baked goods. Or the way emulsifiers stabilize the creamy texture of mayonnaise, preventing oil and vinegar from separating. These are just a few examples of how scientific principles are at work, transforming ingredients into enjoyable meals.
Core Scientific Disciplines Intersecting with Food
The science of food draws upon several core scientific disciplines. These disciplines provide the foundational knowledge necessary to understand the complexities of food.
- Chemistry: Understanding the chemical composition of food, the reactions that occur during processing and cooking, and the properties of food components like carbohydrates, proteins, and fats. For instance, the breakdown of starch into sugars during baking is a chemical reaction that affects the texture and sweetness of bread.
- Biology: Examining the biological processes involved in food production, including the growth of plants and animals, the role of microorganisms in fermentation and spoilage, and the impact of genetics on food characteristics. Consider the use of yeast in bread-making, a biological process that produces carbon dioxide, causing the dough to rise.
- Physics: Applying physical principles to understand food processing techniques, such as heat transfer, mass transfer, and fluid dynamics. For example, the way heat is conducted through a steak during grilling affects its internal temperature and doneness.
- Engineering: Designing and optimizing food processing equipment and systems, ensuring efficient and safe food production. This includes areas like food packaging, preservation, and the development of new food products.
- Nutrition: Studying the relationship between food and health, including the role of nutrients in the body, the impact of diet on disease, and the development of dietary guidelines. This involves analyzing the nutritional content of food and understanding how the body utilizes it.
Chemistry’s Role in Culinary Delights: Food The Delicious Science
The world of food is fundamentally shaped by chemistry, a science that dictates everything from the flavors we savor to the textures we experience. Understanding the chemical reactions that occur during cooking allows us to appreciate the transformation of ingredients and to control the final outcome of a dish. From the browning of a perfectly seared steak to the airy lightness of a soufflé, chemistry is the unseen architect of culinary masterpieces.
Browning Reactions and Flavor Development
Browning in cooking is a critical process, primarily driven by two key chemical reactions: the Maillard reaction and caramelization. These reactions are responsible for the complex flavors and aromas that develop during cooking, contributing significantly to the appeal of many dishes.The Maillard reaction, named after chemist Louis-Camille Maillard, is a non-enzymatic browning reaction that occurs between amino acids and reducing sugars at elevated temperatures.
This complex series of reactions produces hundreds of different flavor compounds, contributing to the savory notes in cooked foods. The specific flavors produced depend on the amino acids and sugars present, as well as the cooking temperature and time.Caramelization, on the other hand, is the oxidation of sugar. It occurs when sugars are heated to high temperatures, resulting in the formation of various compounds that give foods a characteristic brown color and a sweet, sometimes slightly bitter, flavor.
This process is responsible for the distinctive taste of caramelized onions or the golden crust of a crème brûlée.
The Maillard reaction: amino acids + reducing sugars → flavor compounds
These reactions can be influenced by several factors:
- Temperature: Higher temperatures generally accelerate both the Maillard reaction and caramelization. However, excessive heat can lead to burning and undesirable flavors.
- pH: The acidity or alkalinity of a food can affect the rate and type of reactions.
- Moisture: The presence of water can influence the reaction rate; too much moisture can inhibit browning.
- Type of Sugar/Amino Acid: Different sugars and amino acids will yield different flavor profiles. For instance, the Maillard reaction with fructose produces a more intense flavor than with glucose.
Examples of foods that greatly benefit from these browning reactions include:
- Seared Steaks: The Maillard reaction is responsible for the flavorful crust on a seared steak. The amino acids in the meat proteins react with the sugars present, creating the desirable brown color and savory taste.
- Baked Bread: The golden-brown crust on bread is a result of the Maillard reaction and caramelization of sugars in the dough. This process contributes to the bread’s aroma and flavor.
- Caramelized Onions: The slow cooking of onions at a moderate temperature allows for caramelization of the natural sugars, resulting in a sweet and complex flavor profile.
Fats and Their Impact on Food Texture
Fats play a crucial role in determining the texture of food. The type of fat used, whether saturated, unsaturated, or a combination, influences how a dish feels in the mouth, from the crispness of a fried potato to the creamy richness of a sauce. Understanding the properties of different fats allows for greater control over culinary outcomes.Fats are primarily composed of triglycerides, which consist of a glycerol molecule and three fatty acids.
The fatty acids determine the physical properties of the fat, such as its melting point and its behavior at different temperatures.
- Saturated Fats: These fats have no double bonds in their fatty acid chains, making them solid at room temperature. They contribute to a firm texture and are often used in baked goods to provide structure. Examples include butter and coconut oil.
- Unsaturated Fats: These fats contain one or more double bonds in their fatty acid chains, making them liquid at room temperature. They contribute to a softer texture and are often used in sauces and dressings. Examples include olive oil and canola oil. Unsaturated fats can be further classified into monounsaturated and polyunsaturated fats.
- Trans Fats: These fats are created through the process of hydrogenation, where hydrogen is added to unsaturated fats to make them more solid. They are generally less healthy than saturated fats and can contribute to a waxy texture.
The impact of fat on food texture can be observed in various dishes:
- Baked Goods: Saturated fats, like butter, create a flaky texture in pastries and a tender crumb in cakes by interfering with gluten development and providing structure.
- Fried Foods: The type of fat used for frying affects the crispness and texture. Fats with higher smoke points, such as vegetable oil, are preferred for deep-frying, as they can withstand higher temperatures without breaking down.
- Sauces: Unsaturated fats, like olive oil, can create a smooth and creamy texture in sauces. The emulsification of oil and water is a key element in the consistency of many sauces.
Enzymes in Food Preservation and Manipulation
Enzymes are biological catalysts that speed up chemical reactions in food. They play a critical role in both food preservation and the modification of food properties. Understanding the behavior of enzymes allows for controlling these reactions to extend shelf life and enhance flavor or texture.Enzymes can cause both desirable and undesirable changes in food. In some cases, they are beneficial, such as in the fermentation of yogurt.
In other cases, they can lead to spoilage, such as the browning of cut fruits.Enzymes can be manipulated in several ways:
- Temperature Control: Heating foods can denature enzymes, rendering them inactive. This is a common method of food preservation, such as pasteurization. Conversely, cold temperatures can slow down enzymatic reactions, extending shelf life.
- pH Adjustment: Changing the acidity or alkalinity of a food can affect enzyme activity. Some enzymes are more active at specific pH levels. For instance, the addition of lemon juice to apples prevents browning.
- Inhibition: Certain substances can inhibit enzyme activity. For example, the addition of salt can slow down the activity of some enzymes.
- Oxygen Control: Limiting exposure to oxygen can prevent enzymatic reactions that require oxygen, such as the browning of fruits and vegetables.
Examples of enzyme manipulation:
- Fruit Preservation: The browning of cut fruits is caused by the enzyme polyphenol oxidase (PPO). To prevent this, fruits can be blanched (briefly heated), treated with an acid (such as lemon juice), or stored in a low-oxygen environment.
- Cheese Making: Enzymes, such as rennet, are used to coagulate milk proteins in cheese production. The type and concentration of enzymes used affect the final texture and flavor of the cheese.
- Fermentation: Enzymes in yeast convert sugars into alcohol and carbon dioxide, which is essential for making bread and alcoholic beverages. The control of temperature and other conditions helps to control this enzymatic process.
Physics in the Kitchen
The application of physics principles is fundamental to understanding and mastering cooking techniques. From the way heat transfers to the effects of pressure, physics dictates the outcomes of culinary processes. Understanding these principles allows for greater control over food preparation and the ability to achieve desired results consistently.
Heat Transfer Principles in Cooking
Heat transfer is crucial in cooking, influencing how food cooks and the final texture. There are three primary modes of heat transfer: conduction, convection, and radiation.Conduction involves heat transfer through direct contact. For example, when a pan is placed on a stovetop, the heat from the burner transfers to the pan and then to the food in contact with the pan.
The efficiency of conduction depends on the material; metals like copper and aluminum are excellent conductors, while materials like wood are poor conductors.Convection involves heat transfer through the movement of fluids (liquids or gases). In a pot of boiling water, the heated water rises, transferring heat to the cooler water above, which then sinks to be heated. This creates a circular motion, ensuring even cooking.
Similarly, in an oven, heated air circulates, cooking food from all sides.Radiation involves heat transfer through electromagnetic waves. This is how a broiler or a grill cooks food. Infrared radiation emitted from the heat source transfers energy directly to the food, causing it to cook. The distance from the heat source affects the intensity of radiation and, consequently, the cooking speed.
Pressure’s Effect on Cooking Times and Pressure Cookers
Pressure significantly affects cooking times, particularly in pressure cookers. Increased pressure raises the boiling point of water. At sea level, water boils at 212°F (100°C). However, inside a pressure cooker, the increased pressure can raise the boiling point to 250°F (121°C) or higher.
The relationship between pressure and boiling point is described by the Clausius-Clapeyron equation, which shows that an increase in pressure results in an increase in the boiling point.
This higher temperature allows food to cook much faster. For instance, dried beans, which can take hours to cook on a stovetop, can be cooked in a pressure cooker in a fraction of the time. The increased pressure also forces moisture into the food, resulting in a tender and often more flavorful result.Pressure cookers operate by creating a sealed environment.
As the food heats, steam builds up inside, increasing the pressure. A regulator valve controls the pressure, preventing it from exceeding a safe level. The elevated temperature and pressure accelerate the cooking process, making pressure cookers efficient tools for preparing a variety of dishes.
Experiment: Impact of Cooling Methods on Food Texture
The rate at which food cools impacts its final texture. This experiment demonstrates the effects of different cooling methods on a cooked food item, such as a custard or a poached egg.The experiment requires the following materials:
- A batch of the food item (e.g., custard or poached eggs) prepared identically.
- Two or more containers, one large enough to hold an ice bath.
- Ice.
- Water.
- Thermometers (at least two).
- Timer.
Procedure:
- Prepare the food item according to a standard recipe. Divide the cooked food into equal portions.
- Prepare an ice bath in one container by filling it with ice and water. Ensure the ice bath is thoroughly mixed and at a consistently low temperature.
- Place one portion of the food item into the ice bath. Record the initial temperature of the food item and the temperature of the ice bath.
- Place another portion of the food item at room temperature. Record its initial temperature.
- Monitor the temperature of both portions using the thermometers at regular intervals (e.g., every minute) for a set duration (e.g., 15 minutes). Record the temperatures.
- Observe and document the changes in texture and appearance of both portions during and after the cooling process.
Expected Results:The food item cooled in the ice bath will cool much faster than the one at room temperature. The rapid cooling in the ice bath can affect the texture. For example, a custard cooled rapidly may have a smoother texture than one cooled slowly, which may develop a slightly grainy texture due to continued cooking. The poached egg cooled rapidly will stop cooking quickly and remain more tender, while the egg at room temperature will continue cooking for a longer time.
This experiment demonstrates the impact of the cooling rate on the food’s final characteristics.
The Biology of Taste and Texture
The experience of eating is a complex interplay of senses, with taste and texture playing pivotal roles in our enjoyment of food. These sensory experiences are not isolated; they are intricately linked and work in concert with other senses, such as smell, to create the overall perception of flavor. This section will explore the biological mechanisms underlying taste and texture, demonstrating how these sensory aspects contribute to our culinary experiences.
Taste Receptor Contribution to Flavor Perception
Taste perception relies on specialized receptors located primarily on the tongue, but also found in other areas of the oral cavity. These receptors detect different taste qualities, which then combine with other sensory inputs to form the complete flavor profile.The primary taste qualities are:
- Sweet: Sweetness is typically associated with sugars, such as glucose and fructose. Sweet receptors are activated by the binding of these molecules to specific receptor proteins.
- Sour: Sourness is caused by the presence of acids, such as citric acid found in lemons. Acidic substances release hydrogen ions (H+), which activate sour receptors.
- Salty: Saltiness is detected by sodium ions (Na+), which are crucial for nerve and muscle function. Sodium chloride (NaCl), common table salt, activates these receptors.
- Bitter: Bitterness is often associated with potentially harmful substances. There are many different bitter receptors, each capable of detecting a variety of bitter compounds.
- Umami: Umami, often described as savory or meaty, is triggered by the presence of glutamate, an amino acid found in foods like aged cheese and mushrooms. Glutamate binds to specific receptors, creating the umami sensation.
Each taste receptor sends signals to the brain, which interprets these signals and combines them with information from other senses to create the overall flavor experience. For instance, the combination of sweet and sour, as found in a lemon tart, creates a complex and balanced flavor profile.
Food Texture and Scientific Basis
Food texture is a critical aspect of the eating experience, influencing both the physical sensation and the overall flavor perception. Texture is determined by the physical structure of the food and how it interacts with the mouth. The following table details common food textures and the scientific principles behind them.
Texture | Structural Basis | Scientific Explanation | Example |
---|---|---|---|
Crisp | Presence of air pockets and a rigid structure. | When a crisp food is bitten, the structure fractures readily, creating a sharp breaking sound and sensation. The air pockets allow for easy breakage. | Potato chips |
Chewy | Network of interconnected molecules that resist deformation. | The food requires significant force to break down, creating a prolonged sensation in the mouth. Proteins like gluten in wheat products contribute to this texture. | Gummy candy |
Smooth | Small particle size and uniform distribution of components. | The food glides easily over the tongue with minimal friction. The absence of large particles prevents a rough or grainy sensation. | Custard |
Creamy | Emulsification of fat and water with small fat globules. | The fat globules provide a lubricating effect, creating a rich and velvety mouthfeel. The emulsion is stabilized by proteins or emulsifiers. | Ice cream |
The manipulation of these structural elements through cooking and food processing allows for the creation of a vast array of textures, contributing to the diverse culinary experiences we enjoy.
The Interplay of Mouth and Nose in Flavor Creation
Flavor is a multi-sensory experience, with taste and smell working together in a complex interaction. The mouth provides the initial taste sensations, while the nose contributes significantly to the overall flavor perception.The process involves:
- Chewing: As food is chewed, it releases volatile aroma compounds.
- Retro-nasal Olfaction: These aroma compounds travel through the back of the mouth and up into the nasal cavity, where they are detected by olfactory receptors. This is known as retro-nasal olfaction.
- Taste and Aroma Integration: The brain combines the taste signals from the tongue with the aroma signals from the nose to create the overall flavor perception.
The aroma of food can significantly influence how we perceive its taste. For example, if a strawberry is eaten while holding the nose, only the basic tastes (sweetness, sourness) will be detected. However, when the nose is released, the aroma of the strawberry floods the nasal cavity, adding the characteristic strawberry flavor to the overall experience. This demonstrates the crucial role of the nose in creating the full flavor profile of food.
In essence, flavor is the integration of taste and smell, with texture adding another layer of complexity to the overall experience.
Food Preservation: Extending Freshness
Food preservation is a critical aspect of food science, playing a vital role in ensuring food safety, extending shelf life, and reducing food waste. By understanding and applying scientific principles, we can inhibit microbial growth, slow down enzymatic reactions, and prevent physical and chemical changes that lead to food spoilage. This allows us to enjoy a wider variety of foods throughout the year and across geographical locations.
Scientific Principles of Food Preservation
Several scientific principles underpin common food preservation methods. These methods aim to control the factors that cause food spoilage, such as microbial growth, enzymatic activity, and oxidation.
- Canning: Canning involves heating food to a temperature that destroys spoilage microorganisms and enzymes, followed by sealing the food in airtight containers. The key principle is heat sterilization.
- Heat Sterilization: The high heat, typically achieved through boiling or pressure cooking, denatures proteins and inactivates enzymes present in the food, and most importantly, destroys the microorganisms (like bacteria, yeasts, and molds) that cause spoilage.
The effectiveness of heat sterilization depends on the type of food, the size and shape of the container, and the initial microbial load.
- Airtight Seal: Sealing the container prevents recontamination by microorganisms from the environment. The vacuum created during cooling further inhibits microbial growth and prevents oxygen from reacting with the food, which can cause spoilage and off-flavors.
- Heat Sterilization: The high heat, typically achieved through boiling or pressure cooking, denatures proteins and inactivates enzymes present in the food, and most importantly, destroys the microorganisms (like bacteria, yeasts, and molds) that cause spoilage.
- Freezing: Freezing slows down or stops microbial growth and enzymatic reactions by lowering the temperature.
- Temperature Reduction: Freezing water to ice crystals inhibits the growth of microorganisms and slows down the rate of chemical reactions, including enzymatic activity that causes food deterioration.
- Crystal Formation: The rate of freezing affects the size of ice crystals. Rapid freezing creates smaller ice crystals, which cause less damage to the food’s cellular structure compared to slow freezing, which results in larger ice crystals. This preserves the texture of the food better.
- Pickling: Pickling involves preserving food in an acidic solution, typically vinegar.
- Acidity: The high acidity of the pickling solution (low pH) inhibits the growth of most spoilage microorganisms. Most bacteria cannot survive in highly acidic environments.
- Osmosis: The pickling process often involves osmosis, where water moves out of the food and into the brine, leading to a firm texture. Salt and other ingredients also contribute to the flavor and preservation.
- Dehydration: Removing water from food prevents microbial growth, as microorganisms require water to survive and multiply.
- Water Activity: Dehydration reduces the water activity (aw) of food. Water activity is the amount of unbound water available for microbial growth. Reducing the water activity below a certain level inhibits microbial activity.
- Concentration: The concentration of sugars and salts in the food increases during dehydration, which further inhibits microbial growth and can enhance flavor.
Homemade Pickles: A Step-by-Step Guide, Food the delicious science
Making homemade pickles is a practical application of food preservation principles. The process involves several steps, each designed to inhibit microbial growth and ensure the pickles’ safety and shelf life.
- Selection and Preparation of Vegetables: Choose fresh, firm vegetables like cucumbers. Wash them thoroughly to remove any dirt or debris. Trim the ends of the cucumbers, as these may contain enzymes that can soften the pickles.
- Brine Preparation: The brine is the pickling solution. A typical brine consists of water, vinegar (usually white vinegar with 5% acidity), salt (pickling salt, which is pure and doesn’t contain iodine), and spices (such as dill, garlic, and peppercorns).
- Vinegar: The vinegar provides the acidity (low pH) that inhibits the growth of spoilage microorganisms.
- Salt: Salt helps to draw water out of the vegetables through osmosis, which helps to firm the pickles. It also contributes to the flavor and acts as a preservative.
- Spices: Spices add flavor and can also have antimicrobial properties.
- Packing the Jars: Pack the vegetables into sterilized jars, leaving some headspace at the top. Add the brine, ensuring the vegetables are completely submerged. Remove air bubbles.
- Processing (Canning): The jars must be processed using the water bath canning method to ensure that they are safe for long-term storage.
- Water Bath Canning: Place the filled jars in a water bath canner, ensuring they are covered with water. Bring the water to a boil and process for the recommended time, which depends on the type of food, the size of the jars, and the altitude.
This heat treatment destroys any remaining spoilage microorganisms and creates a vacuum seal.
- Vacuum Seal: During cooling, the air inside the jars contracts, creating a vacuum seal. This prevents recontamination and extends the shelf life of the pickles.
- Water Bath Canning: Place the filled jars in a water bath canner, ensuring they are covered with water. Bring the water to a boil and process for the recommended time, which depends on the type of food, the size of the jars, and the altitude.
- Cooling and Storage: After processing, remove the jars from the canner and allow them to cool completely. Check the seals. Store the pickles in a cool, dark place. Properly canned pickles can last for several months to a year.
Packaging and Shelf Life
The packaging materials and methods significantly influence a food’s shelf life. The primary goal of packaging is to protect the food from spoilage factors, such as oxygen, moisture, light, and microorganisms.
- Packaging Materials:
- Glass: Glass jars are commonly used for canning because they are airtight, non-reactive, and provide a good barrier against oxygen and moisture.
- Plastic: Plastic containers are used for a variety of foods. The barrier properties of the plastic depend on the type of plastic. Some plastics are excellent barriers to oxygen and moisture, while others are not.
- Metal Cans: Metal cans are airtight and protect food from light, oxygen, and moisture. They are often used for canned goods.
- Flexible Packaging: Flexible packaging, such as pouches and films, is lightweight and can be customized to provide specific barrier properties.
- Packaging Methods:
- Modified Atmosphere Packaging (MAP): MAP involves changing the composition of the air inside the package to extend shelf life. This is often achieved by removing oxygen and replacing it with gases like nitrogen or carbon dioxide. This method is particularly effective for fresh produce.
- Vacuum Packaging: Vacuum packaging removes air from the package, which reduces oxygen levels and inhibits microbial growth and oxidation. This method is commonly used for meats and cheeses.
- Aseptic Packaging: Aseptic packaging involves sterilizing the food and the packaging separately, then combining them in a sterile environment. This method extends shelf life without the need for refrigeration.
The Science of Baking: A Rising Success
Baking, at its core, is a fascinating blend of chemistry and physics, transforming simple ingredients into delectable treats. From the airy lightness of a croissant to the satisfying chew of a sourdough loaf, the science behind baking dictates the texture, flavor, and appearance of the final product. Understanding the intricate processes involved allows for greater control and precision in the kitchen, leading to consistently successful results.
The Role of Gluten in Bread Making
Gluten, a protein complex formed from the combination of glutenin and gliadin found in wheat flour, plays a pivotal role in bread making. Its unique properties are responsible for the structure and texture of bread.Gluten’s function in bread making involves several key aspects:
- Structure: When water is added to wheat flour and the dough is kneaded, gluten develops, forming a network of interconnected strands. This network traps the gases produced during fermentation, allowing the bread to rise and creating its characteristic airy texture. Think of it as a scaffolding that supports the entire structure.
- Elasticity and Extensibility: Gluten provides both elasticity and extensibility. Elasticity allows the dough to spring back when stretched, while extensibility allows it to be stretched without tearing. This balance is crucial for trapping gases and achieving the desired volume.
- Texture: The amount of gluten development affects the final texture. Over-kneading can lead to a tough bread, while under-kneading can result in a dense loaf. The type of flour also influences gluten development; bread flour, with its higher protein content, develops more gluten than cake flour.
- Flavor: Gluten indirectly contributes to flavor development. During fermentation, gluten proteins are broken down by enzymes, releasing amino acids that contribute to the complex flavors of bread.
Comparing and Contrasting Leavening Agents
Leavening agents are essential for creating the rise and airy texture in baked goods. They work by producing gases, primarily carbon dioxide (CO2), which become trapped within the dough or batter, causing it to expand. Different leavening agents operate through distinct mechanisms, each suited for specific baking applications.Here’s a comparison of common leavening agents:
- Yeast: Yeast is a living microorganism that feeds on sugars in the dough, producing CO2 as a byproduct of fermentation. This process also creates other flavor compounds. Yeast requires time and warmth to activate and is typically used in breads and other baked goods that require a slow rise. The reaction can be summarized as:
C6H12O6 (sugar) + Yeast → 2CO2 (carbon dioxide) + 2C2H5OH (ethanol)
The ethanol evaporates during baking.
- Baking Soda (Sodium Bicarbonate): Baking soda is an alkaline compound that requires an acidic ingredient to react and release CO
2. Common acidic ingredients include buttermilk, vinegar, and lemon juice. The reaction is rapid, making baking soda suitable for quick breads and cakes. The reaction is:NaHCO3 (baking soda) + Acid (e.g., vinegar) → CO2 (carbon dioxide) + H2O (water) + Sodium salt
The speed of the reaction makes it important to bake items containing baking soda immediately after mixing.
- Baking Powder: Baking powder is a complete leavening agent containing both an acid and a base (baking soda). It can be single-acting or double-acting. Single-acting baking powder releases CO2 upon contact with moisture, while double-acting baking powder releases some CO2 when mixed and the rest when heated. Double-acting baking powder is more common as it allows for some flexibility during the baking process.
The Maillard Reaction in Baking
The Maillard reaction is a complex series of chemical reactions between amino acids and reducing sugars that occurs at high temperatures, such as during baking. This reaction is responsible for the browning of baked goods, as well as the development of desirable flavors and aromas.The Maillard reaction involves a multi-step process:
- Initial Reaction: The reaction begins when the carbonyl group of a reducing sugar (such as glucose or fructose) reacts with the amino group of an amino acid (from proteins). This forms an unstable intermediate.
- Rearrangement and Degradation: The unstable intermediate undergoes a series of complex rearrangements and degradations, forming various flavor compounds, including aldehydes, ketones, and heterocyclic compounds. These compounds contribute to the characteristic flavors of baked goods.
- Polymerization: The reaction also leads to the formation of melanoidins, which are brown pigments that contribute to the browning of the crust. The amount of browning depends on the temperature, pH, and the type of ingredients used.
The Maillard reaction is influenced by several factors:
- Temperature: Higher temperatures accelerate the reaction, which is why baking at the correct temperature is essential for browning and flavor development. For example, a loaf of bread baked at a higher temperature will develop a crispier crust.
- pH: The reaction proceeds more rapidly at a slightly alkaline pH.
- Moisture: The reaction occurs optimally at a moderate moisture level.
- Type of Sugars and Amino Acids: Different sugars and amino acids react at different rates, leading to a wide range of flavors and aromas.
Flavor Interactions: Building Deliciousness
The art of cooking transcends mere ingredient combination; it’s a symphony of flavor interactions. Understanding how different flavor compounds mingle and react is crucial for creating dishes that are more than the sum of their parts. These interactions, often complex and nuanced, determine the overall taste experience, from the initial burst of flavor to the lingering aftertaste.
Flavor Compound Synergy
Flavor compounds, the molecules responsible for taste and aroma, don’t exist in isolation. They interact with each other, often in ways that amplify or modify their individual characteristics. This synergy is what creates complex and nuanced flavors.
- Additive Effects: Some flavors simply add to each other, creating a more intense overall taste. For instance, combining the sweetness of strawberries with the tartness of balsamic vinegar results in a balanced and more complex flavor profile.
- Suppression: Certain flavor compounds can suppress or mask others. For example, the bitterness of some vegetables can be masked by the addition of fat or sugar. This is why chefs often use fat to balance bitter flavors.
- Synergistic Enhancement: This is where the magic happens. When certain flavors combine, they create a taste that is greater than the sum of their individual parts. A classic example is the combination of tomatoes and basil. Both have distinct flavors, but when combined, they create a more vibrant and complex flavor profile than either ingredient alone. The umami compounds in tomatoes and the volatile oils in basil contribute to this synergistic effect.
- Flavor Pairing: Flavor pairing principles, often based on the idea that ingredients that share key flavor compounds will complement each other, are widely used to design recipes.
Flavor Pairing Principles in Recipe Design
Flavor pairing principles offer a framework for recipe development, guiding chefs and home cooks in creating harmonious flavor combinations.
- Shared Aroma Compounds: This is a core principle. Ingredients that share similar volatile aroma compounds are likely to pair well. For example, chocolate and coffee both contain pyrazines, which contribute to their roasted and nutty flavors. This explains why chocolate and coffee are often paired.
- Matching Flavor Profiles: Ingredients with similar overall flavor profiles, such as sweet, sour, bitter, salty, and umami, can complement each other. For example, the sweetness of fruit often pairs well with the acidity of vinegar or citrus.
- Contrasting Flavors: Sometimes, opposites attract. Pairing ingredients with contrasting flavors can create a balanced and exciting dish. The combination of sweet and salty, such as in caramel and sea salt, is a prime example.
- Regional Cuisines as Inspiration: Studying traditional cuisines from around the world can provide valuable insights into successful flavor pairings. Regional dishes have evolved over time, often based on locally available ingredients and cultural preferences, leading to time-tested combinations.
A Complex Dish’s Flavor Profile: A Blockquote
Consider a dish of pan-seared scallops with a lemon-butter sauce, served over a bed of saffron risotto and garnished with crispy capers.
The scallops provide a delicate, sweet, and slightly briny base flavor. The Maillard reaction during searing creates savory compounds that add depth. The lemon-butter sauce introduces bright acidity and richness, with the butter’s fat molecules coating the tongue, enhancing the perception of flavor. The saffron risotto offers an earthy and slightly floral flavor, with the saffron’s crocin compounds contributing a subtle bitterness that balances the richness of the dish. The capers provide a burst of salty and tangy flavor, their capsaicin contributing a pleasant bite that cuts through the richness and sweetness.
The synergy of these flavors is key. The sweetness of the scallops is complemented by the acidity of the lemon and the saltiness of the capers. The butter in the sauce helps to round out the flavors, creating a cohesive and balanced experience. The saffron risotto adds complexity and depth, while the capers provide a textural and flavor contrast. This combination of flavors, textures, and aromas creates a truly delicious and memorable dish.
Food and Health: The Nutritional Connection
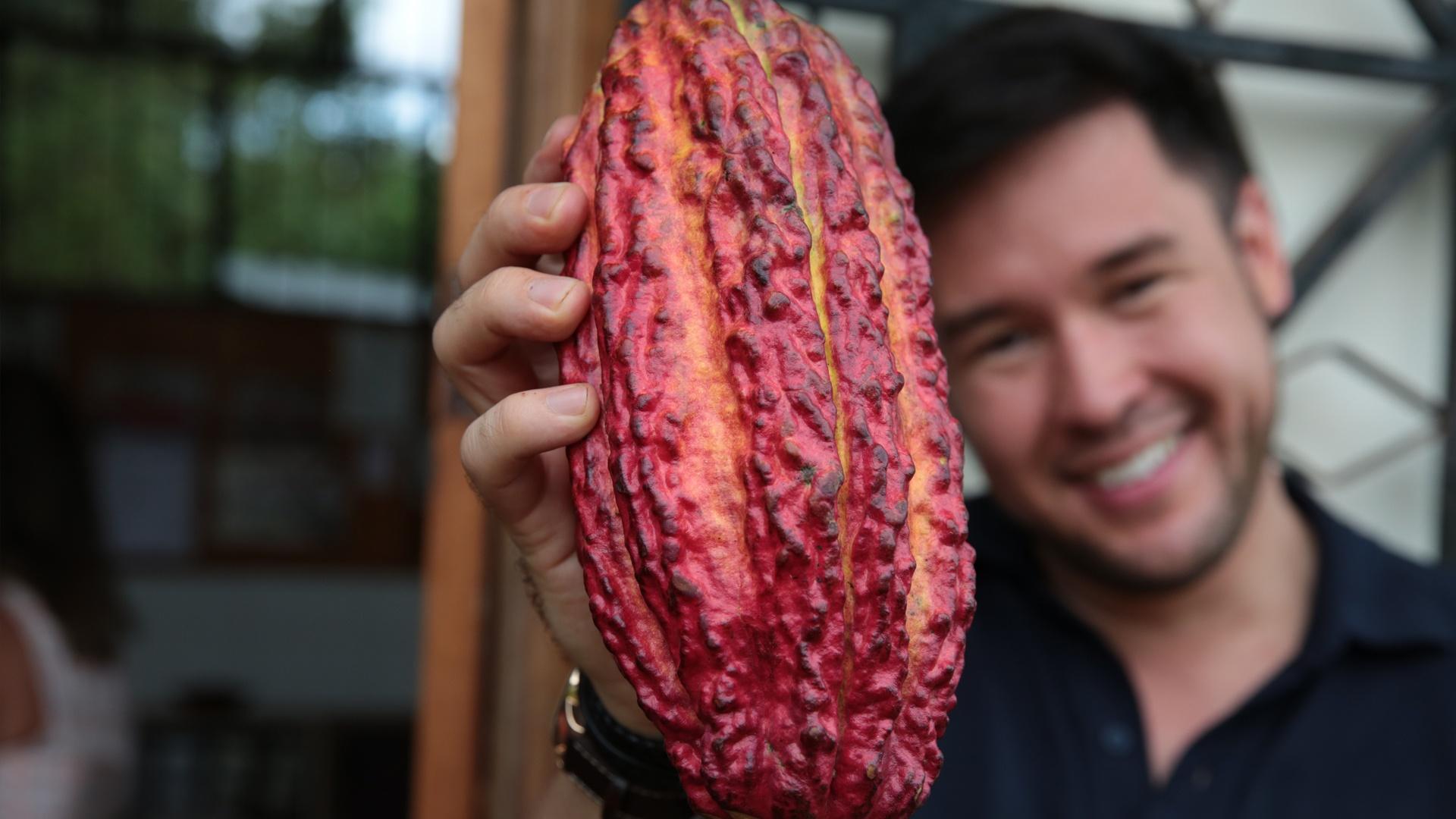
The relationship between food and health is a cornerstone of human well-being, and understanding the science behind this connection is crucial for making informed dietary choices. From the impact of cooking methods on nutrient availability to the intricacies of food allergies and intolerances, a deep dive into this topic reveals how the food we consume profoundly influences our health.
Impact of Cooking Methods on Nutrient Content
Cooking significantly alters the nutrient composition of food, sometimes enhancing and sometimes diminishing the nutritional value. The specific impact depends on the cooking method, the food type, and the nutrients involved.* Heat and Water-Soluble Vitamins: Boiling and steaming can lead to the leaching of water-soluble vitamins, such as Vitamin C and B vitamins, into the cooking water. This is because these vitamins are easily dissolved in water.
The longer the cooking time, the more significant the loss.
Fat-Soluble Vitamins and Minerals
Fat-soluble vitamins (A, D, E, and K) and minerals are generally more stable during cooking. However, high-heat methods, such as frying, can lead to some degradation of these nutrients, especially if the cooking oil is overheated.
Protein
Cooking denatures proteins, changing their structure and making them easier to digest. However, overcooking can lead to protein breakdown and loss of amino acids.
Fiber
Some cooking methods, such as boiling, can soften fiber, making it easier to digest. However, prolonged cooking can also break down some of the fiber content.
Antioxidants
Certain cooking methods can either increase or decrease the availability of antioxidants. For example, cooking tomatoes can increase the bioavailability of lycopene, an antioxidant. However, some antioxidants can be destroyed by heat.
Example
Steaming Vegetables
Steaming preserves more nutrients, especially water-soluble vitamins, compared to boiling, because the vegetables are not submerged in water.
Grilling Meats
Grilling can lead to the formation of heterocyclic amines (HCAs) and polycyclic aromatic hydrocarbons (PAHs), which are potentially carcinogenic compounds.
Macronutrients and Their Roles
Macronutrients are the essential nutrients required in large amounts to provide energy and support bodily functions. They are broadly categorized into carbohydrates, proteins, and fats.* Carbohydrates:
Primary energy source for the body.
Provide glucose, which is used by cells for fuel.
Include sugars, starches, and fiber.
Examples
Grains, fruits, vegetables, and legumes.
Proteins
Essential for building and repairing tissues, enzymes, and hormones.
Composed of amino acids.
Provide a secondary energy source when carbohydrates and fats are insufficient.
Examples
Meat, poultry, fish, eggs, dairy products, beans, and nuts.
Fats
Provide concentrated energy.
Help absorb fat-soluble vitamins.
Support cell growth and development.
Include saturated, unsaturated, and trans fats.
Examples
Oils, nuts, seeds, and fatty animal products.
Water
Essential for almost every bodily function.
Transports nutrients, regulates body temperature, and aids in waste removal.
Often overlooked, but crucial for overall health.
For descriptions on additional topics like chinese food in clifton nj, please visit the available chinese food in clifton nj.
Food Allergies and Intolerances
Food allergies and intolerances are distinct conditions that involve adverse reactions to certain foods. Understanding the underlying mechanisms helps differentiate between them.* Food Allergies:
An immune system response to a specific food protein, mistakenly identified as harmful.
Involves the production of immunoglobulin E (IgE) antibodies.
Symptoms can range from mild (hives, itching) to severe (anaphylaxis).
Common allergens include peanuts, tree nuts, milk, eggs, soy, wheat, fish, and shellfish.
Treatment involves avoiding the allergen and, in severe cases, carrying epinephrine (an EpiPen).
Food Intolerances
A digestive system reaction to a food or food component.
Does not involve the immune system.
Symptoms are usually less severe than those of allergies and may include bloating, gas, diarrhea, or abdominal pain.
Common intolerances include lactose intolerance (inability to digest lactose, a sugar found in milk) and gluten intolerance (sensitivity to gluten, a protein found in wheat, barley, and rye).
Treatment involves avoiding or limiting the offending food. –
Example
Lactose Intolerance
Individuals with lactose intolerance lack sufficient lactase, the enzyme needed to break down lactose. This results in undigested lactose passing into the colon, where it is fermented by bacteria, leading to gas, bloating, and other digestive symptoms.
Celiac Disease
A severe form of gluten intolerance where the immune system attacks the small intestine in response to gluten. This can damage the villi (small finger-like projections) that absorb nutrients, leading to malnutrition and other health problems.
Modern Food Technologies: Innovations in Eating
The realm of food science constantly evolves, driven by technological advancements that reshape how we produce, process, and consume food. These innovations aim to enhance food safety, extend shelf life, improve nutritional value, and cater to evolving consumer preferences. This section explores some of the most impactful modern food technologies, examining their scientific underpinnings and societal implications.
Genetically Modified Foods: Benefits and Drawbacks
Genetically modified (GM) foods, also known as genetically engineered (GE) foods, represent a significant technological advancement in agriculture. The process involves altering the genetic material of plants or animals to introduce desirable traits, such as pest resistance or enhanced nutritional content. However, the technology also raises complex ethical and environmental considerations.
- Benefits of Genetically Modified Foods: GM foods offer several advantages, contributing to both agricultural efficiency and consumer health.
- Increased Crop Yields: Crops engineered for pest resistance, like Bt corn, require fewer pesticides, leading to higher yields. For example, Bt corn produces its own insecticide, reducing the need for external sprays. This translates to more food production per acre of land.
- Enhanced Nutritional Value: Golden Rice, engineered to produce beta-carotene (a precursor to Vitamin A), addresses vitamin A deficiency in regions where rice is a dietary staple. This is a significant example of how GM technology can improve public health.
- Herbicide Tolerance: Crops like Roundup Ready soybeans are engineered to tolerate herbicides, allowing farmers to control weeds more effectively. This can reduce the need for tilling, which helps conserve soil.
- Extended Shelf Life: Some GM crops, such as Flavr Savr tomatoes, are engineered to have a longer shelf life, reducing food waste. This can be achieved by slowing down the ripening process.
- Disease Resistance: GM crops can be engineered to resist various diseases, such as the papaya ringspot virus, saving the Hawaiian papaya industry.
- Drawbacks of Genetically Modified Foods: Despite their potential, GM foods also face concerns regarding their environmental impact and potential health effects.
- Environmental Concerns: The widespread use of herbicide-tolerant crops can lead to increased herbicide use, potentially impacting biodiversity and contributing to the development of herbicide-resistant weeds. The overuse of herbicides can also affect soil health and water quality.
- Potential for Allergenicity: Introducing new genes into crops could potentially introduce new allergens. Thorough testing is required to assess the allergenicity of GM foods before they are released for consumption.
- Unintended Effects: Modifying the genetic makeup of crops can sometimes lead to unintended consequences, such as changes in the nutritional composition or the production of unexpected compounds.
- Impact on Biodiversity: The widespread adoption of GM crops can reduce the diversity of crop varieties, making agricultural systems more vulnerable to pests and diseases. Monoculture farming, often associated with GM crops, can lead to a loss of biodiversity.
- Ethical Concerns: Some people have ethical concerns about modifying the genetic makeup of food, questioning the naturalness of GM foods.
Food Irradiation: The Science of Preservation
Food irradiation is a preservation technique that exposes food products to ionizing radiation to kill microorganisms, insects, and parasites, thereby extending shelf life and enhancing food safety. The process does not make the food radioactive. The scientific basis lies in the ability of radiation to damage the DNA of these organisms, preventing them from reproducing and causing spoilage or illness.
- The Scientific Basis of Food Irradiation: Food irradiation utilizes three main sources of ionizing radiation: gamma rays (from cobalt-60 or cesium-137), X-rays, and electron beams. These forms of radiation have enough energy to break chemical bonds within the cells of microorganisms, leading to their inactivation.
- Gamma Rays: These are highly penetrating and are effective for treating a wide range of food products, including spices, fruits, and vegetables.
- X-rays: These are produced by accelerating electrons to high speeds and then bombarding them with a metal target. They are also highly penetrating.
- Electron Beams: These are generated by electron accelerators and are particularly effective for treating the surface of food products.
The amount of radiation absorbed by the food is measured in kilograys (kGy). The dose is carefully controlled to achieve the desired effect while ensuring the food remains safe and palatable. For instance, spices might be irradiated at a dose of 10 kGy to reduce microbial contamination, while fruits and vegetables may receive lower doses.
- Role in Food Safety: Food irradiation plays a critical role in enhancing food safety by reducing the risk of foodborne illnesses.
- Eliminating Pathogens: Irradiation effectively kills harmful bacteria, such as
-Salmonella*,
-E. coli*, and
-Listeria*, which can cause serious food poisoning. This is particularly important for foods like poultry, meat, and seafood. - Extending Shelf Life: By reducing the microbial load, irradiation extends the shelf life of perishable foods, reducing food waste. For example, irradiated strawberries can last significantly longer than non-irradiated ones.
- Controlling Insects and Parasites: Irradiation is effective in controlling insects and parasites that can infest food products, ensuring their safety and quality. This is especially important for imported foods.
- Reducing Spoilage: Irradiation can slow down the ripening process in fruits and vegetables, reducing spoilage and preserving their quality.
- Eliminating Pathogens: Irradiation effectively kills harmful bacteria, such as
Futuristic Food Concept: Nutrient-Optimized 3D-Printed Meals
A futuristic food concept involves the creation of nutrient-optimized, personalized meals using 3D printing technology. This concept leverages advances in materials science, food chemistry, and digital fabrication to produce food tailored to an individual’s specific nutritional needs and preferences.
- Scientific Principles Behind the Production: The process would involve several key scientific principles.
- Nutritional Analysis and Personalization: Individuals would undergo nutritional assessments, including blood tests and dietary analysis, to determine their specific nutrient requirements (e.g., protein, vitamins, minerals). This data would be used to create personalized dietary profiles.
- Food Formulation and Material Science: Scientists would formulate food inks or cartridges composed of various ingredients, including proteins (e.g., plant-based proteins, cultured meat), carbohydrates, fats, vitamins, and minerals. These inks would be designed to have specific textures, flavors, and nutritional profiles. The inks would be designed to have specific rheological properties, such as viscosity and flow behavior, to ensure they can be extruded and printed accurately.
- 3D Printing Technology: A 3D printer would use a layer-by-layer approach to build the meal from the food inks. Different nozzles would dispense different ingredients to create complex structures and textures. The printer would be programmed to follow a digital design based on the individual’s nutritional profile and desired meal.
- Flavor Encapsulation and Enhancement: Flavor compounds could be encapsulated within microcapsules to control their release and enhance the sensory experience. This would allow for precise flavor control and the creation of complex flavor profiles.
- Precision Cooking and Texture Control: The 3D printer could incorporate cooking elements, such as microwave or infrared heaters, to cook the food during the printing process. This would allow for precise control over the cooking process and the creation of specific textures. For example, a meal might have a seared exterior and a tender interior.
- Example Scenario: A person with iron deficiency could have a 3D-printed meal designed to provide a specific dose of iron, formulated with ingredients that enhance iron absorption (e.g., vitamin C). The meal might be printed with a specific texture and flavor profile based on the individual’s preferences, making it both nutritious and enjoyable. A senior citizen with swallowing difficulties could receive a meal with a specific texture to ease consumption.
- Real-World Applications and Potential: The concept has the potential to revolutionize food production, catering to diverse needs and preferences.
- Personalized Nutrition: Individuals could receive meals tailored to their specific health conditions, dietary restrictions, and athletic performance goals.
- Reduced Food Waste: The ability to print meals on demand could minimize food waste by producing only what is needed.
- Sustainable Food Production: The use of alternative protein sources and precision agriculture could reduce the environmental impact of food production.
- Space Exploration: 3D food printing could be essential for providing astronauts with nutritious and customized meals during long-duration space missions.
Food and the Senses: A Multi-Sensory Experience
The enjoyment of food is far more complex than simply satisfying hunger. It’s a deeply sensory experience, engaging all five senses in a symphony of pleasure. From the visual appeal of a beautifully plated dish to the satisfying crunch of a crispy texture, each sensory element contributes to our overall perception and appreciation of a meal. Understanding how these senses interact allows us to consciously enhance the dining experience.
Sensory Elements Contributing to Meal Enjoyment
Our perception of food is a complex interplay of sight, sound, smell, taste, and touch. Each sense provides unique information that influences our overall enjoyment. The brain integrates these inputs, creating a holistic experience.
Visual, Auditory, and Tactile Elements of a Dish
The following table details the visual, auditory, and tactile elements often present in a dish, alongside examples of how they manifest. These elements work together to shape our initial impressions and ongoing experience of the food.
Sensory Element | Description | Example | Impact on Experience |
---|---|---|---|
Visual | The appearance of the food, including color, shape, and arrangement. | A vibrant salad with colorful vegetables arranged artistically, a perfectly browned steak with grill marks. | Creates initial expectations, influences appetite, and signals freshness and quality. A visually appealing dish is often perceived as more appetizing. |
Auditory | The sounds associated with the food, such as crunching, sizzling, or popping. | The crackle of a freshly baked baguette, the sizzle of bacon frying, the crunch of a potato chip. | Adds to the anticipation and pleasure of eating. Certain sounds are associated with specific textures and can indicate freshness or doneness. |
Tactile | The texture of the food in the mouth, including sensations like smooth, crunchy, chewy, or creamy. | The velvety texture of a mousse, the crispness of a fried chicken skin, the chewiness of a steak. | Provides crucial information about the food’s consistency and mouthfeel. Texture significantly impacts overall enjoyment and satisfaction. |
Enhancing the Dining Experience Using Sensory Elements
By consciously considering and manipulating these sensory elements, we can significantly enhance the dining experience. This involves paying attention to presentation, cooking techniques, and ingredient choices.
- Visual Enhancement: Consider plating techniques, color combinations, and the use of garnishes to create a visually appealing dish. For example, a simple plate of pasta can be transformed by adding a sprinkle of fresh herbs, a drizzle of olive oil, and a dusting of Parmesan cheese.
- Auditory Enhancement: Focus on cooking methods that create appealing sounds. Sizzling, crackling, and popping sounds can be very enticing. Consider the sounds the food makes as you eat it.
- Tactile Enhancement: Combine different textures to create a more complex and satisfying experience. The juxtaposition of smooth and crunchy elements, for instance, can add excitement. A dish of creamy risotto with crispy fried onions exemplifies this principle.
- Smell and Taste: While not explicitly covered in the table, the olfactory and gustatory senses are critical. Use fragrant herbs and spices, and consider the balance of flavors to create a delicious and memorable meal.
Wrap-Up
In conclusion, food the delicious science provides a compelling look at the science that goes into our food. It showcases how understanding these principles can elevate our culinary skills and enhance our appreciation for the meals we enjoy. By exploring the roles of chemistry, physics, biology, and more, we gain a deeper understanding of how flavors are created, textures are achieved, and the sensory experience of eating is heightened.
Embracing the science behind our food unlocks a world of culinary possibilities, transforming the everyday act of eating into a journey of discovery and delight.