The food chain deep sea is a fascinating world of survival, where life thrives in the perpetual darkness and crushing pressure of the ocean’s depths. Unlike surface ecosystems that depend on sunlight, deep-sea life relies on a unique energy source: chemosynthesis. This process, carried out by specialized bacteria, uses chemicals from hydrothermal vents and other sources to create energy, forming the base of a complex food web that supports a diverse array of organisms.
This intricate system demonstrates nature’s resilience and adaptability, offering a glimpse into the remarkable strategies employed by creatures to survive in one of Earth’s most extreme environments. From the tiny bacteria to the colossal predators, each organism plays a crucial role in this underwater ecosystem, contributing to the delicate balance of life in the deep sea.
Introduction to the Deep Sea Food Chain
The deep sea, a realm of perpetual darkness and immense pressure, harbors a unique and fascinating food web. This intricate network of life, far removed from the sun’s life-giving rays, presents extraordinary challenges and adaptations. Understanding the deep-sea food chain is crucial for appreciating the resilience and diversity of life on Earth.
Fundamental Principles of a Food Chain: Energy Flow
A food chain illustrates the flow of energy and nutrients through an ecosystem. It is a linear sequence of organisms where each organism consumes the one below it, thereby transferring energy. This energy transfer follows the laws of thermodynamics, specifically the second law, which dictates that with each transfer, some energy is lost as heat.The fundamental components of a food chain are:
- Producers: These organisms, often plants or algae, convert energy from the sun (in most ecosystems) or chemicals (in chemosynthetic ecosystems) into organic matter through photosynthesis or chemosynthesis. This organic matter serves as the base of the food chain.
- Consumers: These organisms obtain energy by consuming other organisms. They are categorized based on their diet:
- Primary Consumers (Herbivores): Eat producers.
- Secondary Consumers (Carnivores/Omnivores): Eat primary consumers.
- Tertiary Consumers (Carnivores/Omnivores): Eat secondary consumers, and so on.
- Decomposers: These organisms, such as bacteria and fungi, break down dead organic matter (detritus), releasing nutrients back into the environment to be used by producers.
Energy flow is typically represented as:
Producer → Primary Consumer → Secondary Consumer → Tertiary Consumer → Decomposer
This illustrates the direction of energy transfer, with arrows indicating “is eaten by.”
Unique Challenges of the Deep Sea Environment
The deep sea environment presents a host of extreme conditions that significantly impact the survival and evolution of its inhabitants. These challenges necessitate remarkable adaptations.The primary challenges include:
- Absence of Sunlight: The deep sea is perpetually dark, eliminating the possibility of photosynthesis as a primary energy source for most of the ecosystem.
- Extreme Pressure: Pressure increases dramatically with depth. Organisms must withstand crushing forces, requiring specialized cellular structures and biochemical adaptations. For instance, many deep-sea fish have swim bladders filled with oil rather than gas to cope with the pressure.
- Low Temperatures: Water temperatures in the deep sea are consistently cold, typically just above freezing. Organisms must have enzymes that function effectively at these low temperatures.
- Limited Food Availability: The deep sea receives very little organic matter from the surface waters, making food scarce. This scarcity necessitates efficient foraging strategies and adaptations for surviving long periods without food.
- Oxygen Availability: While oxygen is present, its concentration can vary. Some areas may experience oxygen minimum zones, posing a challenge for respiration.
Primary Producers in Deep-Sea Ecosystems
Due to the absence of sunlight, the deep sea lacks the photosynthetic producers found in surface ecosystems. Instead, deep-sea ecosystems often rely on chemosynthesis. Chemosynthesis uses chemical energy, typically from the oxidation of inorganic compounds like hydrogen sulfide, to produce organic matter.The primary producers in deep-sea ecosystems are:
- Chemosynthetic Bacteria: These bacteria thrive near hydrothermal vents and cold seeps, where chemicals like hydrogen sulfide, methane, and ammonia are released from the Earth’s crust. They oxidize these chemicals to create energy, which is then used to convert carbon dioxide into organic compounds.
- Chemosynthetic Archaea: Similar to bacteria, archaea also perform chemosynthesis, playing a vital role in energy production within these unique environments.
- Symbiotic Relationships: Some organisms, like tube worms, harbor chemosynthetic bacteria within their tissues. The bacteria provide the host with organic nutrients, while the host provides a stable environment and the necessary chemicals for chemosynthesis. For example, the giant tube worm,
-Riftia pachyptila*, found near hydrothermal vents, lacks a mouth and gut. Instead, it relies on chemosynthetic bacteria living within its trophosome, a specialized organ.The worm absorbs hydrogen sulfide and oxygen from the vent water, which the bacteria use to produce food.
These chemosynthetic producers form the base of the deep-sea food chain, supporting a diverse array of consumers.
The Base of the Deep Sea Food Chain
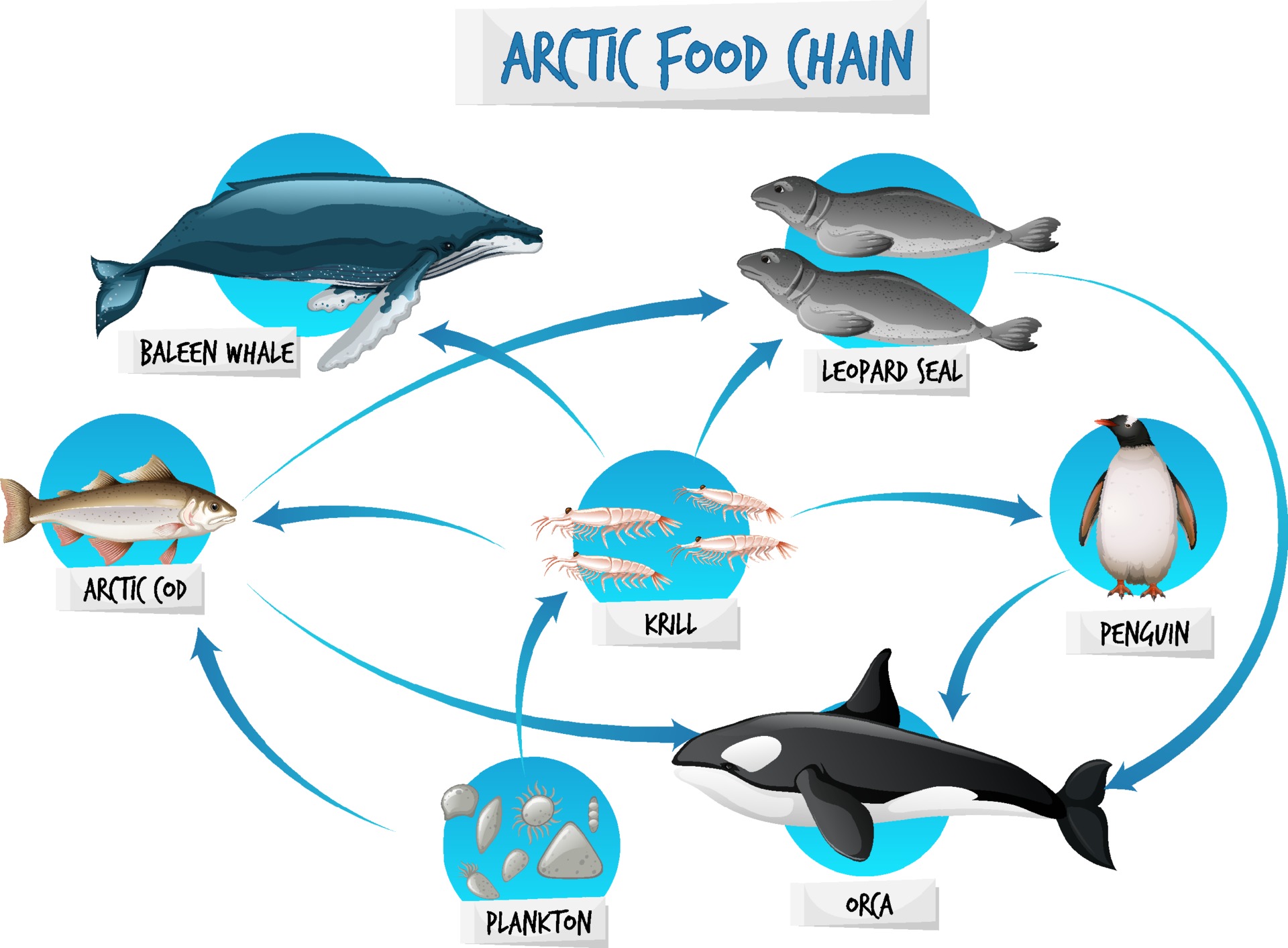
The deep sea, a realm perpetually shrouded in darkness, presents a unique challenge to life. Sunlight, the primary energy source for most ecosystems, is absent. Instead, the foundation of the deep-sea food chain relies on a remarkable process called chemosynthesis, a process that harnesses chemical energy to produce food. This is a stark contrast to photosynthesis, which drives life in sunlit environments.
Chemosynthesis Process and Its Significance
Chemosynthesis is the biological conversion of one or more carbon molecules (usually carbon dioxide or methane) and nutrients into organic matter using the oxidation of inorganic molecules (such as hydrogen sulfide) or methane as a source of energy, rather than sunlight. This process is critical in deep-sea environments because it provides the primary energy source for a wide variety of organisms.
Without chemosynthesis, the complex ecosystems found in hydrothermal vents, cold seeps, and other deep-sea habitats could not exist.
Chemosynthetic Bacteria and Chemical Compounds
Various types of bacteria are involved in chemosynthesis, each specializing in utilizing different chemical compounds. These bacteria are often found in symbiotic relationships with other organisms, such as tube worms and mussels, providing them with nourishment.
- Sulfur-oxidizing bacteria: These bacteria are the most common type found in hydrothermal vent communities. They oxidize hydrogen sulfide (H 2S) released from the vents, converting it into elemental sulfur or sulfate. The energy released from this oxidation is then used to fix carbon dioxide into organic compounds.
H2S + O 2 → S + H 2O + Energy
- Methane-oxidizing bacteria (methanotrophs): Found in cold seeps, these bacteria utilize methane (CH 4) as an energy source. They oxidize methane, often in conjunction with sulfate reduction, to produce energy and organic matter.
CH4 + O 2 → CO 2 + H 2O + Energy
- Ammonia-oxidizing bacteria: These bacteria oxidize ammonia (NH 3) to nitrite (NO 2-), generating energy in the process. They play a crucial role in the nitrogen cycle in certain deep-sea environments.
NH3 + O 2 → NO 2– + H+ + Energy
- Iron-oxidizing bacteria: These bacteria can oxidize ferrous iron (Fe 2+) to ferric iron (Fe 3+), a process that releases energy. They are found in environments where iron is abundant, such as around hydrothermal vents.
Fe2+ + O 2 → Fe 3+ + Energy
Chemosynthetic Communities and Energy Sources
Different chemosynthetic communities thrive in specific locations, depending on the availability of chemical compounds. The table below showcases the variety of these communities and their primary energy sources.
Community Type | Location | Primary Energy Source | Examples of Organisms |
---|---|---|---|
Hydrothermal Vents | Mid-ocean ridges, volcanic areas | Hydrogen Sulfide (H2S) | Giant tube worms (Riftia pachyptila), vent mussels, vent shrimp |
Cold Seeps | Continental margins, areas with methane release | Methane (CH4), Hydrogen Sulfide (H2S) | Mussels, tubeworms, various chemosynthetic bacteria |
Whale Falls | Ocean floor, near whale carcasses | Decomposing whale tissue (releases sulfides and methane) | Bone-eating worms (Osedax), various bacteria |
Subduction Zones | Areas where tectonic plates collide | Methane, Hydrogen Sulfide, and other reduced compounds | Clams, mussels, various chemosynthetic bacteria |
Primary Consumers: The Grazers and Filter Feeders
Primary consumers are the vital link between the base of the deep-sea food web, such as chemosynthetic bacteria or sinking organic matter, and the higher trophic levels. They obtain their energy by feeding directly on these primary producers. Their feeding strategies are incredibly diverse, reflecting the varied resources and conditions found in the deep ocean.
Role of Primary Consumers in the Deep Sea Food Web
Primary consumers are essential for transferring energy from the base of the food web to the rest of the ecosystem. They convert the energy stored in chemosynthetic bacteria or organic matter into a form usable by secondary consumers, such as predators. Their presence influences the distribution and abundance of other organisms in the deep sea. They are also crucial for nutrient cycling, processing organic material and releasing nutrients back into the water.
Without them, the energy flow would be severely limited.
Examples of Deep-Sea Organisms and their Feeding Strategies
Deep-sea primary consumers exhibit a range of adaptations for obtaining food in the challenging environment. Some graze directly on chemosynthetic bacteria around hydrothermal vents, while others filter organic particles from the water column.
- Grazers: These organisms scrape or consume the bacteria directly.
- Examples: Certain species of deep-sea snails and limpets are grazers. They use specialized mouthparts, like a radula (a toothed, tongue-like structure), to scrape the bacterial mats from the surfaces of rocks or vent structures.
- Filter Feeders: These organisms extract organic particles from the water.
- Examples:
- Giant Tube Worms (Riftia pachyptila): These iconic vent organisms possess a plume-like structure that filters hydrogen sulfide and other chemicals from the water. They harbor chemosynthetic bacteria within their bodies, which they provide with these chemicals. In return, the bacteria provide the tube worms with nutrients.
- Bivalves (clams and mussels): Many species of deep-sea bivalves, such as those found near vents or cold seeps, are filter feeders. They use their gills to strain organic particles from the water.
- Some species of amphipods and copepods: These small crustaceans are common filter feeders in the deep sea. They use specialized appendages to capture particles suspended in the water column.
Comparison of Feeding Strategies
The following bullet points highlight the contrasting feeding strategies of different primary consumers.
- Feeding Source: Grazers feed directly on bacterial mats, while filter feeders extract organic particles from the water column.
- Feeding Mechanism: Grazers use scraping or biting mouthparts, while filter feeders employ specialized structures like gills or appendages.
- Habitat: Grazers are often found in close proximity to chemosynthetic environments, while filter feeders can be found in various deep-sea habitats, including vent systems, cold seeps, and the open water column.
- Efficiency: Filter feeders can process large volumes of water, potentially capturing a wider range of organic particles. Grazers have a more direct, localized food source.
- Examples:
- Grazers: Deep-sea snails, limpets.
- Filter Feeders: Giant tube worms, deep-sea bivalves, some crustaceans (amphipods, copepods).
Secondary Consumers and Predators: Navigating the Depths: Food Chain Deep Sea
The deep sea is a realm of perpetual darkness, where life persists through intricate predator-prey relationships. Secondary consumers and predators play a critical role in the deep-sea food web, shaping the distribution and abundance of organisms. These creatures have evolved remarkable adaptations to survive in this extreme environment, relying on specialized senses and hunting strategies to secure their meals.
Types of Secondary Consumers and Predators
The deep sea hosts a diverse array of predators, ranging from small invertebrates to large, specialized fish. These organisms occupy various trophic levels, feeding on primary consumers, other predators, or scavengers.
- Fish: Many fish species are apex predators in the deep sea. Examples include:
- Anglerfish: Known for their bioluminescent lure used to attract prey in the darkness.
- Gulper Eel: Possesses a large mouth and expandable stomach, enabling it to consume prey larger than itself.
- Saber-toothed Viperfish: Features long, needle-like teeth and a bioluminescent photophore, for luring and ambushing smaller fish.
- Invertebrates: Various invertebrates also function as predators. Examples include:
- Giant Squid: A formidable predator that feeds on fish, crustaceans, and other cephalopods.
- Deep-sea Shrimp: Some species are predatory, feeding on smaller crustaceans and other invertebrates.
- Sea Spiders: These arthropods, though small, are predators of other invertebrates, using their proboscis to suck fluids.
- Marine Mammals: While not exclusively deep-sea dwellers, some marine mammals, such as sperm whales, are significant predators in these environments. They hunt squid and other deep-sea creatures.
Adaptations for Hunting in the Dark
Survival in the deep sea necessitates specialized adaptations to overcome the challenges of perpetual darkness. Predators have evolved a variety of sensory and morphological features to detect and capture prey in the absence of sunlight.
- Bioluminescence: Many deep-sea predators use bioluminescence for various purposes:
- Luring Prey: Some species, like anglerfish, have a bioluminescent lure to attract unsuspecting victims. The lure, a modified dorsal fin spine, dangles in front of the fish’s mouth, mimicking a smaller organism.
- Camouflage: Counterillumination is used by some organisms to blend with the faint downwelling light, making them invisible to predators or prey looking up from below.
- Communication: Bioluminescence is used for communication, attracting mates, and signaling other members of their species.
- Sensory Specializations: Predators have enhanced sensory systems to detect prey in the dark.
- Lateral Line System: Many fish possess a lateral line system, a series of sensory organs along their body that detect vibrations in the water. This allows them to sense the movement of prey, even in the absence of light.
- Large Eyes: Some species have large eyes, which are adapted to capture the limited amount of light available in the deep sea. The size of the eye is proportional to the depth, and the greater the depth, the larger the eye.
- Chemoreceptors: Certain predators have developed highly sensitive chemoreceptors to detect chemical signals released by potential prey.
- Morphological Adaptations: Physical characteristics also aid in hunting.
- Large Mouths and Teeth: Many deep-sea predators have large mouths and sharp teeth to capture and hold prey.
- Expandable Stomachs: Some species, like the gulper eel, have expandable stomachs to consume large prey.
- Streamlined Bodies: Some predators possess streamlined bodies to enhance their swimming ability, allowing them to chase prey more efficiently.
Examples of Predator-Prey Relationships
The deep sea is characterized by complex predator-prey interactions. These relationships drive the flow of energy through the ecosystem and influence the structure of deep-sea communities.
- Anglerfish and Small Fish: The anglerfish’s bioluminescent lure attracts smaller fish, which are then ambushed and consumed. The anglerfish’s large mouth and sharp teeth ensure prey capture.
- Giant Squid and Sperm Whales: This is a well-documented predator-prey relationship. Sperm whales dive deep to hunt giant squid, often leaving behind scars from the squid’s tentacles. The whales use echolocation to find their prey in the dark depths.
- Saber-toothed Viperfish and Smaller Fish: The viperfish’s needle-like teeth and ambush hunting style enable it to capture smaller fish. The viperfish uses bioluminescence to attract prey, making it an efficient predator.
The Role of Scavengers and Decomposers
The deep sea, while seemingly barren, teems with life, and the efficiency of its food web relies heavily on the recycling of organic matter. This crucial role is primarily fulfilled by scavengers and decomposers. They are the clean-up crew and the recyclers of the deep, ensuring that nutrients are returned to the system, supporting the continuation of the food web.
Scavengers and Decomposers: Their Vital Function
Scavengers and decomposers are essential to the deep-sea ecosystem. They break down dead organisms, waste products, and other organic materials, returning vital nutrients to the water column and the seafloor. Without their activity, the deep sea would quickly become choked with waste, and the flow of energy through the food web would grind to a halt. These organisms prevent the accumulation of organic debris, and the nutrients released support primary production in the photic zone (where sunlight penetrates), ultimately influencing the entire marine ecosystem.
Types of Scavengers and Decomposers
A variety of organisms play the role of scavengers and decomposers in the deep sea. These include both animals and microorganisms, each with specialized roles in breaking down organic matter.
- Scavengers: These organisms consume dead animals, helping to clear the seafloor. Examples include:
- Deep-sea fish: Many species of fish, such as hagfish and certain species of grenadiers, are opportunistic scavengers. They use their keen senses to locate and consume carcasses.
- Crustaceans: Amphipods, isopods, and certain species of crabs are common scavengers. They are often the first to arrive at a carcass, quickly consuming soft tissues.
- Polychaete worms: Some species of polychaete worms are scavengers, feeding on the remains of dead animals.
- Decomposers: Primarily microorganisms that break down organic matter at a microscopic level. Examples include:
- Bacteria: Bacteria are the primary decomposers in the deep sea. They break down complex organic molecules into simpler substances, releasing nutrients like nitrogen, phosphorus, and carbon. The rate of decomposition is significantly slower in the deep sea due to the cold temperatures and high pressure.
- Fungi: While less abundant than bacteria, fungi also contribute to decomposition, particularly in the breakdown of tougher organic materials.
The Process of Decomposition in the Deep Sea
The process of decomposition in the deep sea is a complex interplay of biological and chemical processes. When an organism dies and sinks to the seafloor, scavengers like amphipods and hagfish will initially consume the soft tissues. Bacteria then colonize the remaining organic material, breaking down the complex molecules through enzymatic reactions. This process releases nutrients like nitrogen and phosphorus back into the water. Some of these nutrients are consumed by chemosynthetic bacteria, which form the base of food chains around hydrothermal vents. Other nutrients are transported by currents and eventually contribute to the productivity of other areas of the ocean. The rate of decomposition is influenced by factors like temperature, pressure, oxygen availability, and the type of organic material. For example, the decomposition of whale falls (the carcasses of whales that sink to the seafloor) can support deep-sea ecosystems for decades, providing a concentrated source of food and nutrients.
Unique Adaptations of Deep-Sea Organisms
The deep sea, a realm of perpetual darkness, extreme pressure, and limited resources, presents a formidable challenge to life. Organisms inhabiting this environment have evolved a remarkable array of adaptations to survive and thrive. These adaptations are crucial for everything from finding food and avoiding predators to reproducing and maintaining internal stability in the face of crushing pressures.
Bioluminescence: A Light in the Darkness
Bioluminescence, the production and emission of light by a living organism, is perhaps the most iconic adaptation of deep-sea creatures. This remarkable ability is employed for a variety of purposes, making it a vital tool for survival in the light-deprived environment.
- Attracting Prey: Many deep-sea predators utilize bioluminescent lures to entice unsuspecting prey. For example, the anglerfish possesses a bioluminescent esca, a modified dorsal fin spine that dangles in front of its mouth, attracting smaller fish. As these smaller fish approach the light, they are ambushed and consumed.
- Camouflage and Counter-illumination: Some organisms, like certain species of squid, employ bioluminescence for counter-illumination. They produce light on their ventral (underside) surface to match the faint downwelling sunlight, effectively camouflaging themselves against the background. This prevents predators from seeing their silhouette against the dimly lit water above.
- Communication and Mating: Bioluminescence is also used for communication, especially during mating rituals. Some species use complex patterns of flashing lights to signal potential mates. The firefly squid, for instance, displays intricate bioluminescent patterns for attracting mates.
- Defense: Certain organisms use bioluminescence as a defense mechanism. Some jellyfish release a burst of bioluminescent light when threatened, startling predators and potentially allowing them to escape. Others may use bioluminescent “bombs” or flashes to distract attackers.
Pressure Resistance and Other Physiological Adaptations
The extreme pressure in the deep sea poses a significant challenge to life. Organisms have evolved various physiological adaptations to withstand these crushing forces. Beyond pressure resistance, they also exhibit other unique traits to cope with the harsh conditions.
- Pressure Resistance: Deep-sea organisms have adapted to withstand immense pressure. This is often achieved through several mechanisms:
- Cellular Structure: The cell membranes of deep-sea organisms are often more flexible and contain a higher proportion of unsaturated fatty acids. This helps maintain membrane fluidity and function under pressure.
- Enzyme Stability: Enzymes, the biological catalysts that drive biochemical reactions, are designed to function efficiently under high pressure. Some deep-sea fish have enzymes that are more pressure-resistant than those found in shallow-water species.
- Absence of Gas-Filled Spaces: Many deep-sea organisms lack gas-filled spaces, such as swim bladders, which are easily compressed by pressure. This prevents the organisms from being crushed.
- Metabolic Adaptations: The scarcity of food in the deep sea has driven the evolution of slower metabolisms. This allows organisms to conserve energy and survive for extended periods between meals. Some deep-sea fish, for instance, have very low metabolic rates.
- Sensory Adaptations: In the absence of sunlight, deep-sea organisms rely heavily on other senses. Many species have evolved highly sensitive sensory systems:
- Lateral Line System: This system detects vibrations in the water, allowing organisms to sense the movement of prey or predators.
- Chemoreceptors: These receptors detect chemicals in the water, aiding in the location of food or potential mates.
- Large Eyes (in some species): Some deep-sea fish have large eyes to maximize the amount of light they can capture. However, not all deep-sea organisms have large eyes; many are blind or have reduced eyes, as the light is often not useful.
- Reproductive Strategies: Due to the difficulty of finding mates in the vast and dark deep sea, some species have developed unique reproductive strategies:
- Hermaphroditism: Some species are hermaphroditic, possessing both male and female reproductive organs. This increases the chances of successful reproduction.
- Bioluminescent Mating Displays: As mentioned earlier, bioluminescence plays a crucial role in attracting mates.
- Pheromone Signaling: Some species use pheromones, chemical signals, to attract mates over long distances.
Vertical Migration and Food Web Connections
The deep sea, despite its remoteness, is not isolated. It’s intricately linked to the surface waters through a fascinating phenomenon known as vertical migration. This daily movement of organisms between the surface and the depths plays a crucial role in the transfer of energy and nutrients, fundamentally shaping the deep-sea food web.
The Mechanics of Vertical Migration
Vertical migration, also known as diel vertical migration (DVM), is a widespread behavior in the ocean. Many organisms, including zooplankton, small fish, and even some larger predators, undertake this daily journey. They spend the daylight hours in the deeper, darker waters to avoid predation, and ascend to the surface at night to feed on phytoplankton and other organisms. This process is driven primarily by light intensity and the availability of food.
- The Role of Light: Light serves as the primary cue for vertical migration. During the day, the intense sunlight at the surface makes organisms vulnerable to visual predators. Therefore, they descend to the deeper, darker waters where they are less visible. At night, when the light diminishes, they migrate upwards.
- Food Availability: The surface waters are rich in phytoplankton, the base of the marine food web. Zooplankton and other primary consumers migrate to the surface at night to feed on these photosynthetic organisms. This feeding activity provides a significant energy source for the migrating organisms.
- Predator Avoidance: The constant threat of predation is a major driver of vertical migration. Smaller organisms use the dark depths as a refuge from predators during the day. Larger predators, such as fish and squid, may also participate in vertical migration, following their prey.
Surface Influence on the Deep Sea
The surface ocean significantly influences the deep-sea ecosystem by providing a continuous supply of organic matter. This transfer of energy and nutrients is essential for sustaining life in the deep sea, where sunlight is absent.
- The Biological Pump: The biological pump is the process by which carbon is transferred from the surface waters to the deep ocean. When phytoplankton die or are consumed by zooplankton, their organic matter sinks to the depths. This “marine snow,” consisting of dead organisms, fecal pellets, and other organic debris, becomes a primary food source for deep-sea organisms.
- Direct Consumption: Migrating organisms that feed at the surface and then descend to the deep sea contribute to the food supply. These organisms are consumed by deep-sea predators, transferring energy from the surface to the depths.
- Nutrient Cycling: The sinking of organic matter also contributes to nutrient cycling in the deep sea. Decomposers break down this organic material, releasing nutrients that can be used by other organisms.
Surface and Deep Sea Food Web Connections Diagram
The following describes a simplified diagram of the connections between the surface and deep-sea food webs. The diagram emphasizes the flow of energy and nutrients from the surface to the deep sea.
Element | Description |
---|---|
Sunlight | The primary energy source for the surface ocean, fueling photosynthesis by phytoplankton. |
Phytoplankton | The base of the surface food web, producing energy through photosynthesis. |
Zooplankton | Primary consumers that feed on phytoplankton, participating in vertical migration. |
Migrating Fish/Squid | Secondary consumers that feed on zooplankton at the surface, descending to deeper waters. |
Marine Snow | Organic matter (dead organisms, fecal pellets) sinking from the surface, providing food for deep-sea organisms. |
Deep-Sea Consumers | Organisms in the deep sea that consume marine snow and migrating organisms. |
Deep-Sea Predators | Apex predators in the deep sea that feed on other deep-sea organisms. |
Decomposers | Bacteria and other organisms that break down organic matter, recycling nutrients in the deep sea. |
Upwelling (Arrow pointing upwards) | Nutrient-rich water rising from the deep ocean to the surface, fueling phytoplankton growth. |
The diagram shows the flow of energy and matter from the sun to phytoplankton, then to zooplankton and other consumers. Some zooplankton and consumers migrate, transferring energy directly to the deep sea. Marine snow also provides food for deep-sea organisms, and decomposers recycle nutrients. Upwelling is included to show how the deep sea contributes to the surface ecosystem as well.
Threats to the Deep Sea Food Chain
The deep sea, once considered largely untouched by human activity, is increasingly facing significant threats. These threats, stemming from a variety of human-induced and global environmental changes, are disrupting the delicate balance of the deep-sea food chain, with potentially devastating consequences for the biodiversity and overall health of these ecosystems. Understanding these threats is crucial for developing effective conservation strategies.
Pollution in the Deep Sea
Pollution poses a considerable threat to deep-sea environments. This pollution encompasses various forms, each impacting the food chain differently.
- Plastic Pollution: Plastic debris, a ubiquitous pollutant, is a significant concern. Plastics, including microplastics, can be ingested by deep-sea organisms, leading to physical harm, such as blockages in digestive tracts, and the introduction of harmful chemicals. For example, studies have found plastic particles in the stomachs of deep-sea fish and invertebrates, demonstrating the widespread impact of plastic pollution even in the most remote areas of the ocean.
- Chemical Pollution: Industrial and agricultural runoff, as well as the release of chemicals from oil spills and other accidents, introduces toxic substances into the deep sea. These pollutants can bioaccumulate in organisms, meaning they become more concentrated as they move up the food chain. This can lead to reproductive problems, developmental issues, and ultimately, a decline in populations of vulnerable species.
Heavy metals, such as mercury and lead, are particularly concerning.
- Noise Pollution: Anthropogenic noise, primarily from shipping, seismic surveys, and military activities, can disrupt the behavior of deep-sea animals. Many deep-sea creatures rely on sound for communication, navigation, and finding food. Noise pollution can mask these essential sounds, interfering with their ability to survive.
Impact of Climate Change
Climate change is dramatically altering the deep sea environment, impacting the food chain in numerous ways.
- Ocean Acidification: The absorption of excess carbon dioxide (CO2) from the atmosphere is causing the ocean to acidify. This acidification makes it harder for organisms, such as corals and shellfish, to build and maintain their shells and skeletons. The effects of ocean acidification extend through the food chain, as organisms at the base of the food chain, such as plankton, are negatively impacted.
- Changes in Sea Surface Temperature: Rising sea surface temperatures affect the deep sea. This can alter the distribution of species, as organisms migrate to find more suitable conditions. Changes in temperature can also affect the sinking of organic matter from the surface, impacting the food supply for deep-sea creatures.
- Deoxygenation: As the ocean warms, it holds less oxygen. This, coupled with increased stratification of the water column, can lead to reduced oxygen levels (hypoxia) or even oxygen-free zones (anoxia) in the deep sea. This severely impacts organisms that require oxygen to survive, such as many fish and invertebrates.
Human Activities and their Effects
Various human activities directly and indirectly impact the deep sea food chain.
- Deep-Sea Mining: The extraction of minerals from the seafloor, a relatively new industry, poses a significant threat. Mining operations can destroy habitats, release sediment plumes that smother organisms, and introduce toxic substances. The disruption of the seafloor can also impact the flow of nutrients and organic matter, disrupting the food chain.
- Fishing: Bottom trawling, a fishing method that drags nets along the seafloor, can devastate deep-sea habitats and remove organisms that form the base of the food chain. Overfishing of specific species can also disrupt the balance of the food web. For instance, the removal of a key predator can lead to an overpopulation of its prey, impacting other species in the food chain.
- Oil and Gas Exploration: The exploration and extraction of oil and gas in the deep sea carry risks of spills and leaks, introducing hydrocarbons into the environment. These pollutants are toxic to many deep-sea organisms and can disrupt the food chain. Even the construction of infrastructure, such as pipelines, can disrupt habitats.
Conservation Efforts for Deep-Sea Protection
Protecting deep-sea environments requires a multifaceted approach, involving various conservation efforts.
For descriptions on additional topics like always food safe com, please visit the available always food safe com.
- Establishing Marine Protected Areas (MPAs): MPAs are designated areas where human activities are restricted or prohibited to protect marine ecosystems. MPAs can help to safeguard vulnerable habitats, protect biodiversity, and allow populations to recover from the impacts of human activities. Effective MPAs are crucial for conserving deep-sea environments.
- Sustainable Fishing Practices: Implementing and enforcing sustainable fishing practices is crucial to minimize the impact of fishing on deep-sea ecosystems. This includes using less destructive fishing gear, setting catch limits, and protecting vulnerable species.
- Regulation of Deep-Sea Mining: Developing and enforcing regulations for deep-sea mining is essential to minimize its environmental impacts. This includes conducting thorough environmental impact assessments before mining operations begin and implementing strict monitoring and mitigation measures.
- Reducing Pollution: Addressing pollution, including plastic and chemical pollution, requires global cooperation and action. This includes reducing plastic production and waste, cleaning up existing pollution, and regulating the release of chemicals into the environment.
- Addressing Climate Change: Mitigating climate change is critical to protect the deep sea from the impacts of ocean acidification, warming, and deoxygenation. This requires reducing greenhouse gas emissions and transitioning to renewable energy sources.
Exploration and Research of Deep Sea Food Webs
The deep sea, a realm of perpetual darkness and extreme pressure, presents significant challenges to scientific exploration. Despite these difficulties, researchers employ various innovative methods and technologies to unravel the complexities of its food webs. This research is crucial for understanding the biodiversity, ecological processes, and the impacts of human activities on this vast and largely unexplored environment.
Methods Used to Study Deep Sea Food Webs
Investigating deep-sea food webs necessitates a combination of direct and indirect approaches. These methods are designed to overcome the logistical and environmental hurdles of the deep ocean.
- Remote Operated Vehicles (ROVs): ROVs are unmanned underwater vehicles tethered to a surface vessel. They are equipped with cameras, lights, and manipulators, allowing scientists to visually survey the seafloor, collect samples, and conduct experiments. These are essential for direct observation of organisms and their interactions.
- Autonomous Underwater Vehicles (AUVs): AUVs are untethered, robotic submarines that can operate independently, following pre-programmed paths. They can map the seafloor, collect data on water parameters, and capture images and video. Their autonomy allows for extended missions in remote areas.
- Submersibles: Manned submersibles allow scientists to directly observe the deep sea environment. These vessels are equipped with life support systems and can reach significant depths, providing a unique perspective for studying the behavior of organisms and the structure of the food web. For example, the
-Alvin* submersible has been instrumental in numerous deep-sea discoveries. - Deep-Sea Trawling: Trawling involves dragging a net along the seafloor to collect organisms. This method provides samples for identifying species, analyzing gut contents, and studying trophic relationships. However, it can be destructive to the habitat.
- Baiting and Trapping: Researchers deploy baited traps or cameras to attract and observe organisms. This technique is particularly effective for studying scavengers and predators. The composition of the bait influences the types of organisms attracted.
- Molecular Techniques: DNA barcoding and stable isotope analysis are used to identify species and trace energy flow through the food web. DNA barcoding identifies species by analyzing short, standardized DNA sequences, while stable isotope analysis reveals the sources of carbon and nitrogen in an organism’s diet.
- Hydroacoustic Surveys: Sonar technology can be used to detect and track the presence of organisms, particularly in the water column. This method is especially useful for studying the vertical migration of organisms and the distribution of biomass.
Examples of Current Research Being Conducted in the Deep Sea, Food chain deep sea
Ongoing research efforts are continually expanding our knowledge of deep-sea ecosystems. These projects are crucial for understanding the dynamics of these complex environments.
- Hydrothermal Vent Communities: Studies focus on the unique food webs that thrive around hydrothermal vents. These vents release chemicals that support chemosynthetic bacteria, forming the base of the food chain. Research examines the interactions between these bacteria and the diverse fauna that depend on them.
- Deep-Sea Coral Reefs: Research explores the biodiversity and trophic structure of deep-sea coral reefs. These reefs provide habitat for numerous organisms and are vulnerable to fishing and climate change. Studies investigate the food web interactions within these ecosystems and their sensitivity to environmental stressors.
- Abyssal Plain Food Webs: Investigations into the food webs of the abyssal plains, the vast, flat areas of the deep ocean, focus on the sources of organic matter that support life in these nutrient-poor environments. Research explores the role of marine snow, scavengers, and the long-term effects of organic matter deposition.
- Deep-Sea Mining Impacts: Studies are being conducted to assess the potential impacts of deep-sea mining on food webs. Mining activities can disrupt habitats, release pollutants, and alter the structure of benthic communities. Research aims to understand these impacts and develop strategies for sustainable resource management.
- Impacts of Plastic Pollution: Research is increasingly focused on the effects of plastic pollution on deep-sea food webs. Plastic debris can be ingested by organisms, accumulate in the food chain, and alter the physical structure of the seafloor.
Technologies Used to Explore and Study Deep-Sea Environments
Advancements in technology have been instrumental in expanding our ability to study the deep sea. These technologies provide unprecedented access to this challenging environment.
- High-Resolution Imaging Systems: These systems, including advanced cameras and sonar, provide detailed images of the seafloor and the organisms that inhabit it. These images are crucial for identifying species, mapping habitats, and observing behavior.
- Advanced Sensors: Sensors measure a wide range of environmental parameters, such as temperature, salinity, pressure, oxygen levels, and the presence of chemicals. These data provide insights into the physical and chemical conditions that shape deep-sea ecosystems.
- Robotics and Automation: ROVs and AUVs are becoming increasingly sophisticated, with improved maneuverability, longer endurance, and the ability to perform complex tasks. These advancements allow for more efficient and extensive exploration.
- DNA Sequencing and Analysis: High-throughput DNA sequencing technologies enable rapid and comprehensive analysis of genetic material, facilitating species identification, understanding of trophic relationships, and assessment of biodiversity.
- Deep-Sea Drills and Corers: These tools are used to collect sediment samples, which provide information about the history of the deep sea, including past climate conditions, the composition of the seafloor, and the presence of fossilized organisms.
- Long-Term Monitoring Stations: These stations are deployed in the deep sea to continuously monitor environmental parameters and biological activity. They provide valuable data on the long-term changes occurring in deep-sea ecosystems.
Closing Summary
In conclusion, the deep-sea food chain is a testament to life’s ability to flourish even in the most challenging conditions. Understanding the intricacies of this ecosystem, from chemosynthesis to predator-prey relationships, is crucial for appreciating the interconnectedness of our planet. As we continue to explore and research the deep sea, it is imperative that we prioritize conservation efforts to protect this fragile and invaluable environment from the threats of pollution and climate change, ensuring its survival for generations to come.