Food can structures form the backbone of the canned food industry, a technology that has revolutionized food preservation and distribution. These seemingly simple containers are complex engineering marvels, designed to protect and preserve food products for extended periods. From their humble beginnings to the sophisticated designs of today, food cans have continuously evolved, driven by the need for efficient, safe, and sustainable food packaging solutions.
The following discussion will dive into the intricacies of food can structures, exploring their components, manufacturing processes, materials, and the critical role they play in ensuring food safety and shelf life.
We’ll delve into the materials that make up these structures, the meticulous processes used to create them, and the coatings that protect both the can and the food inside. We’ll also examine the importance of standardization, the role of sterilization, and the quality control measures that ensure the safety of canned goods. Finally, we’ll explore the environmental impact of food can manufacturing and look at future trends that are shaping the evolution of this essential packaging technology.
Introduction to Food Can Structures
Food can structures are engineered containers designed primarily for the preservation and storage of food products. Their principal function is to provide a barrier against external factors that could compromise the food’s quality, safety, and shelf life. This includes protection from microorganisms, light, oxygen, moisture, and physical damage.
Materials Used in Food Can Construction
The selection of materials is crucial in determining the performance characteristics of food cans. These materials must be inert, non-toxic, and capable of withstanding the sterilization processes used in food preservation.
- Steel: Steel, typically tin-plated steel (tinplate), is the most widely used material. Tinplate offers excellent formability, strength, and corrosion resistance. The tin coating protects the steel from rusting. The type of steel and the thickness of the tin coating are carefully chosen based on the specific food product and the required shelf life.
- Aluminum: Aluminum cans are lighter than steel cans and offer good corrosion resistance, especially when internally coated. They are commonly used for beverages and some food products. Aluminum is also readily recyclable.
- Coatings: Internal coatings are applied to the can’s interior to prevent direct contact between the food and the metal. These coatings are typically made of food-grade polymers, such as epoxy resins or vinyl resins. They provide a barrier against corrosion and prevent the metal from imparting undesirable flavors or colors to the food. External coatings are also used for decoration, labeling, and corrosion protection.
- Seals and Gaskets: The seals and gaskets used in the can’s construction, particularly in the can ends (lids), are crucial for ensuring an airtight and hermetic seal. These seals are typically made of rubber compounds, such as butyl rubber, or other elastomers, which are selected for their sealing properties and resistance to the food product’s contents and processing conditions.
Historical Evolution of Food Can Structures
The development of food can structures reflects advancements in materials science, manufacturing techniques, and food preservation technologies. Early designs were rudimentary, but they have evolved significantly to meet the demands of modern food processing and distribution.
- Early Canning (Early 19th Century): The earliest food cans were made of heavy wrought iron and were sealed by soldering a lid onto the body. These cans were cumbersome, expensive to produce, and prone to corrosion. The initial canning process was primarily used by the military for preserving food supplies.
- Tinplate Introduction (Mid-19th Century): The introduction of tinplate marked a significant improvement. Tinplate cans were lighter, more resistant to corrosion, and could be mass-produced more efficiently. This innovation led to the widespread adoption of canning for commercial food preservation.
- Improvements in Seaming and Sealing (Late 19th and Early 20th Centuries): The development of mechanical seaming machines dramatically improved the speed and reliability of can sealing. Double seam technology, where the lid and body are mechanically interlocked, became the standard. This ensured a hermetic seal, crucial for preventing spoilage.
- Aluminum Cans and Coating Innovations (Mid-20th Century): The introduction of aluminum cans provided a lighter alternative to steel, particularly for beverages. Simultaneously, advancements in internal coatings, such as epoxy resins, enhanced corrosion resistance and food safety.
- Modern Can Designs and Materials (Late 20th Century to Present): Modern food can designs emphasize lightweighting, ease of opening, and sustainability. This includes the use of thinner steel and aluminum, easy-open features (such as pull tabs), and recyclable materials. Research continues to explore new materials and coatings to improve can performance and environmental impact. For instance, the development of “tin-free steel” (TFS) is a move towards reducing reliance on tin, and innovations in polymer-based coatings are focused on enhanced food safety and resistance to specific food types.
Components and Design Elements
The construction of a food can is a carefully engineered process, involving specific components and design choices that ensure the preservation and safety of the contents. These elements work in concert to create a hermetically sealed container, protecting the food from spoilage and maintaining its quality. Understanding these components and their functions is crucial to comprehending the overall effectiveness of food can structures.
Key Components of a Typical Food Can
A typical food can comprises several essential parts, each contributing to its overall functionality. These components are designed to work together, providing a robust and reliable barrier against external factors.
- Body: This is the main cylindrical or rectangular part of the can, which holds the food product. It provides the primary structural support and defines the can’s capacity. The body can be made from various materials, with steel and aluminum being the most common.
- Lid (or End): The lid seals the top of the can, providing a hermetic seal to prevent contamination and leakage. It’s often made from the same material as the body and is designed to be easily opened, depending on the can’s design (e.g., easy-open end).
- Bottom (or End): Similar to the lid, the bottom end seals the can. It’s typically attached in the same manner as the lid, forming a complete seal.
- Seams: These are the critical joints where the body, lid, and bottom are joined together. They are designed to be airtight and watertight, preventing the ingress of air, moisture, and microorganisms. Seams are a vital aspect of can integrity.
Types of Can Bodies and Manufacturing Processes
The manufacturing process for can bodies significantly impacts their structural properties and suitability for different food products. The choice of can body type often depends on factors like cost, production volume, and the specific requirements of the food product.
- Three-Piece Cans: These cans are constructed from three separate pieces: a cylindrical body and two ends (lid and bottom). The body is typically made from a flat sheet of metal that is rolled and welded or soldered along a longitudinal seam to form the cylinder. The ends are then seamed onto the body. This method is cost-effective for large-scale production and allows for a wider range of can sizes and shapes.
The welding or soldering process creates a strong and durable seam.
- Two-Piece Cans: These cans are made from a single piece of metal, usually through a process called drawing and ironing (D&I) or draw and redraw (DRD). In D&I, a metal disc is drawn into a cup, and then the walls are thinned and extended through ironing. DRD involves multiple drawing steps to achieve the desired can depth and shape. The two-piece design eliminates the need for a side seam, resulting in a stronger and more leak-proof can.
Two-piece cans are particularly suitable for beverages and products requiring high internal pressure.
The Role of Can Seams and Sealing Mechanisms
Can seams are critical to the integrity of a food can, ensuring a hermetic seal that prevents spoilage and maintains food safety. The sealing mechanisms employed in can seams are meticulously designed to withstand internal pressures, temperature fluctuations, and handling during transportation and storage.
- Seaming Process: The seaming process involves mechanically interlocking the can body and end flanges. This is achieved using specialized seaming machines that perform multiple operations. The process typically involves curling the flanges, folding them together, and then compressing them to create a tight, airtight seal.
- Types of Seams: The most common type of seam is the double seam, which is used to join the can body and ends. The double seam consists of several folds and interlocking layers of metal, creating a robust seal. There is also the welded side seam in three-piece cans, where the body is welded to create a single cylindrical piece.
- Seal Integrity: The seal’s integrity is maintained by the precise dimensions and tolerances of the seam. Proper seam formation is crucial for preventing leaks and ensuring the can’s ability to withstand internal pressure. Quality control measures, such as seam inspection, are essential to identify and eliminate defective cans.
- Sealing Compounds: Sealing compounds, such as rubber-based compounds, are often applied to the seam to enhance the seal’s effectiveness. These compounds fill any microscopic gaps and provide an additional barrier against leakage.
Basic Food Can Cross-Section Diagram
A cross-section diagram illustrates the internal structure of a food can and its key components. The diagram is essential for understanding the arrangement of the parts and how they work together to create a sealed container.
Diagram Description:
Imagine a vertical cross-section of a typical food can, cut through the lid and body. From top to bottom, the diagram would reveal the following:
- Lid: The top end of the can, showing the metal and the double seam.
- Double Seam (Top): The intricate interlocking of the lid and body flanges, forming a secure seal.
- Headspace: The space between the food product and the lid.
- Food Product: The contents of the can (e.g., vegetables, fruits).
- Can Body: The cylindrical or rectangular main part of the can.
- Double Seam (Bottom): Similar to the top seam, securing the bottom end.
- Bottom: The bottom end of the can, identical in construction to the lid.
Key labels on the diagram would include “Lid”, “Double Seam”, “Can Body”, “Bottom”, and “Food Product”. The diagram would also show the layers of metal folded in the double seam, highlighting the sealing mechanism.
Manufacturing Processes
The creation of food cans involves several sophisticated manufacturing processes, each meticulously designed to ensure the structural integrity, safety, and preservation capabilities of the final product. These processes are highly automated and optimized for mass production, adhering to strict quality control standards. The choice of manufacturing method is heavily influenced by the can’s intended use, the type of food it will contain, and the desired shelf life.
This section details the key manufacturing steps for both three-piece and two-piece can structures, as well as the production of lids and bottoms.
Can Body Fabrication for Three-Piece Cans
Three-piece cans, composed of a body, a top, and a bottom, are a widely used packaging solution. The body of the can is typically constructed from a flat sheet of metal, usually tin-plated steel or aluminum. The manufacturing process involves several key stages:
- Sheet Preparation: The metal sheet is first coated with protective layers, such as a lacquer to prevent corrosion and food contact materials. The sheet is then cut into rectangular blanks, the size depending on the desired can dimensions.
- Body Forming: The rectangular blank is then rolled and formed into a cylindrical shape. This is typically done using a specialized machine that wraps the metal around a mandrel.
- Side Seam Welding/Soldering: The formed cylinder’s edges are joined together to create the side seam. This is the most critical step in the three-piece can manufacturing process. There are two primary methods:
- Welding: High-frequency welding is a common technique. The edges of the can body are brought together, and an electrical current is passed through them, generating heat that fuses the metal.
The process creates a strong and hermetic seal.
- Soldering: Historically, soldering was a primary method. Molten solder, typically a tin-lead alloy, is applied to the seam to join the edges. While effective, soldering is less environmentally friendly due to the lead content and is being phased out in many regions.
- Welding: High-frequency welding is a common technique. The edges of the can body are brought together, and an electrical current is passed through them, generating heat that fuses the metal.
- Flanging: After the side seam is formed, the ends of the cylindrical body are flanged outwards. This creates a lip to which the can ends (lids and bottoms) will be seamed. The flanging process is done using specialized machinery.
- Internal Coating and Decoration: The interior of the can body is often coated with a protective lacquer to prevent the food from reacting with the metal. The exterior can be decorated with printed labels or designs.
Methods for Manufacturing Two-Piece Cans
Two-piece cans, composed of a body and a single end, offer advantages in terms of material usage and structural integrity. The manufacturing methods primarily used are draw and redraw (DRD) and draw and iron (D&I).
- Draw and Redraw (DRD): This method is primarily used for producing cans with a relatively uniform wall thickness.
- Drawing: A circular blank of metal is drawn through a die using a punch. This process forms the initial cup shape.
- Redrawing: The cup is then passed through a series of dies, each of which further reduces the diameter and increases the height of the can body. Each redraw operation reduces the diameter and increases the height of the can.
- Trimming and Flanging: After the final redraw, the can is trimmed to the desired height, and the top edge is flanged to receive the can end.
- Draw and Iron (D&I): This method is commonly used for producing cans with thinner walls and a more uniform wall thickness.
- Drawing: Similar to DRD, a circular blank is drawn into a cup.
- Ironing: The cup is then passed through a series of ironing rings. These rings compress the can walls, thinning them and elongating the can body. The bottom of the can retains its original thickness.
- Trimming and Flanging: The can is trimmed to the required height, and the top edge is flanged.
Lid and Bottom Manufacturing Process
The manufacturing of lids and bottoms, also known as can ends, is a critical step in the overall can production process. These components must provide a secure and hermetic seal to preserve the food inside.
- Material Preparation: Similar to can bodies, can ends are typically made from tin-plated steel or aluminum. The metal sheets are coated with protective lacquers and printing if needed.
- Stamping: The metal sheets are stamped using dies to create the desired shape and features of the can end. This process includes forming the countersink (the recessed area that provides strength), the curl (the rolled edge that facilitates seaming), and any necessary vents or openings.
- Sealing Compound Application: A sealing compound, usually a rubber-based material, is applied to the curl of the can end. This compound creates a hermetic seal when the can end is seamed to the can body.
- Curing: The sealing compound is then cured to ensure its proper sealing properties.
- Decoration and Inspection: The can ends may be decorated with printed labels or designs. They are then thoroughly inspected for defects, ensuring they meet the required quality standards.
Step-by-Step Procedure for a Basic Food Can Production Line
A typical food can production line is highly automated, with each step carefully orchestrated to ensure efficiency and quality. This Artikel details a basic production line.
- Material Feed: Metal sheets (tinplate or aluminum) are fed into the production line.
- Blanking/Slitting: The metal sheets are cut into blanks for two-piece cans or prepared for three-piece can body fabrication.
- Body Forming (for three-piece cans): Metal blanks are rolled and welded or soldered into cylindrical bodies.
- Drawing/Redrawing or Drawing/Ironing (for two-piece cans): Cups are drawn, and then further processed using DRD or D&I methods.
- Flanging: The can bodies (three-piece and two-piece) have their ends flanged.
- Internal Coating: The interior of the can bodies receives a protective coating.
- External Decoration: The exterior of the can bodies is decorated (printing or labeling).
- Can End Production: Metal sheets are stamped, sealing compound is applied, and can ends are cured.
- Seaming: Can ends are seamed onto the can bodies.
- Testing and Inspection: Cans are inspected for leaks, dimensional accuracy, and other quality parameters. This can include pressure testing to simulate the internal pressure during sterilization.
- Packaging and Shipping: The finished cans are packaged and prepared for shipment to food processing facilities.
Materials and Properties: Food Can Structures
The selection of materials is crucial in food can manufacturing, significantly impacting the can’s performance, food preservation, and overall shelf life. The chosen materials must withstand the rigorous processes of food processing, sterilization, and handling, while also ensuring the safety and quality of the packaged food. Understanding the properties of these materials is essential for designing effective and safe food can structures.
Steel Properties in Food Can Construction
Steel, particularly low-carbon steel, is the predominant material used in food can manufacturing due to its strength, formability, and cost-effectiveness. Steel’s properties are tailored through composition and processing to meet the specific demands of food preservation.The following are key properties of steel used in food can construction:
- Tensile Strength: The ability of the steel to resist being pulled apart. This is critical for withstanding the internal pressure generated during thermal processing and the handling of the filled cans. High tensile strength ensures the can maintains its structural integrity.
- Ductility: The capacity of steel to be deformed without fracturing. This allows for the steel to be shaped into the can’s form through drawing, stamping, and other manufacturing processes. Sufficient ductility prevents cracking during these operations.
- Corrosion Resistance: The steel’s resistance to degradation from interaction with the food contents. Steel is typically coated to provide corrosion resistance. The coating protects the steel from reacting with acidic or salty foods, preventing the formation of rust and maintaining the food’s quality.
- Weldability: The ease with which the steel can be welded to create a hermetic seal. Welded seams are a crucial part of can construction, and the steel’s weldability is essential for ensuring a leak-proof container.
- Formability: The ease with which the steel can be shaped without breaking or cracking. This property allows the steel to be formed into the various can components, such as the body, ends, and lids.
Tinplate vs. Tin-Free Steel
Two primary types of steel are utilized in food can production: tinplate and tin-free steel (TFS). Both offer advantages and disadvantages, influencing their application in different canning scenarios.
- Tinplate: Tinplate is steel coated with a thin layer of tin. Tin provides excellent corrosion resistance and acts as a sacrificial anode, protecting the steel from rust if the coating is scratched or damaged. Tinplate is favored for its superior corrosion resistance and solderability, particularly for acidic foods. The tin coating also provides a bright, attractive finish. However, tinplate is more expensive than TFS due to the cost of tin.
- Tin-Free Steel (TFS): TFS, or Electrolytic Chromium Coated Steel (ECCS), has a chromium coating, usually followed by an organic coating. TFS is a cost-effective alternative to tinplate, offering good corrosion resistance, especially for less aggressive food products. TFS is more resistant to sulfide staining, which can occur with tinplate when canning certain sulfur-containing foods. The organic coating can also be customized for specific food applications.
The choice between tinplate and TFS depends on the specific food product, the required shelf life, and the cost considerations. For example, highly acidic foods like tomatoes or fruits often require tinplate, while less corrosive products like vegetables may be packaged in TFS.
Alternative Materials in Food Can Manufacturing
While steel remains the dominant material, alternative materials are also employed, often in specialized applications or to meet specific consumer or environmental demands.
- Aluminum: Aluminum is lightweight and offers good corrosion resistance, especially when anodized or coated. It is commonly used for easy-open ends and some can bodies. Aluminum’s recyclability is a significant advantage, contributing to its growing popularity. Aluminum cans are also favored for their aesthetic appeal and branding opportunities.
- Composite Cans: These cans combine materials like paperboard, aluminum foil, and plastic. Composite cans are typically used for dry foods, snacks, and products that do not require high-temperature sterilization. They offer a cost-effective option and are often chosen for their recyclability and sustainability attributes.
- Glass: Although not a metal, glass jars are also used in food preservation. Glass is inert, offering excellent barrier properties and transparency, allowing consumers to see the product. Glass is ideal for products that require high-temperature processing and is completely recyclable.
The selection of alternative materials is based on factors like the type of food, the desired shelf life, processing requirements, and consumer preferences. For example, aluminum cans are common for beverages because they are lightweight and chill quickly.
Material Properties and Shelf Life
The material properties of food cans directly influence the shelf life of the canned food. The primary function of the can is to provide a barrier against external factors that can degrade the food.
- Corrosion Resistance: The ability of the can to resist corrosion is critical for maintaining food quality and preventing spoilage. Corrosion can lead to the breakdown of the can and the introduction of contaminants into the food.
- Barrier Properties: The can must effectively prevent the ingress of oxygen, light, and moisture. These factors can cause food spoilage through oxidation, discoloration, and loss of nutrients. The barrier properties of the can are directly related to the material’s ability to prevent these elements from reaching the food.
- Hermetic Seal: A perfect seal is essential to prevent the entry of microorganisms. The seal must withstand pressure and temperature changes during processing and storage.
- Strength and Durability: The can must withstand the stresses of processing, handling, and storage without damage. Damage to the can can compromise the seal and allow spoilage.
For example, a can with poor corrosion resistance might allow the steel to rust, which can perforate the can and lead to spoilage. Similarly, a can with a weak seal might allow microorganisms to enter, leading to food spoilage. In contrast, a well-constructed can with appropriate material properties can maintain the food’s quality and safety for an extended period, often several years.
The shelf life is often tested using accelerated shelf-life testing methods, which involve storing the cans at elevated temperatures to simulate longer storage periods. These tests help determine the expected shelf life under various conditions.
Can Coatings and Liners
The application of coatings and liners is a critical aspect of food can manufacturing, serving to protect both the can itself and, crucially, the food product it contains. These protective layers act as a barrier, preventing the interaction of the food with the can material, and shielding the can from the corrosive effects of the food contents. They are essential for maintaining food safety, preserving product quality, and extending shelf life.
Purpose of Internal Can Coatings and Liners
Internal can coatings and liners serve several vital functions. They are designed to protect the can from corrosion caused by the food product, which can lead to the formation of rust and potentially contaminate the food. These coatings also prevent the migration of metal ions from the can material into the food, which could alter its taste, color, and nutritional value.
Furthermore, they provide a barrier against the absorption of flavor and odor from the food into the can material, preserving the original characteristics of the product.
Types of Coatings and Their Applications
A variety of coating types are employed in food can manufacturing, each suited to specific applications based on the food product and processing methods.
- Epoxy Coatings: Epoxy coatings are widely used due to their excellent chemical resistance, flexibility, and adhesion properties. They are particularly effective for acidic foods, such as fruits, vegetables, and tomato-based products. These coatings are applied as a liquid and then cured through a heating process, forming a hard, protective layer. One common example is bisphenol A (BPA)-based epoxy resins.
- Acrylic Coatings: Acrylic coatings offer good flexibility and resistance to a range of food products. They are often used as an alternative to epoxy coatings, especially where BPA concerns exist. Acrylics provide good adhesion and are suitable for a variety of foods, including those with moderate acidity.
- Polyester Coatings: Polyester coatings are known for their good flexibility and resistance to staining. They are often employed for general-purpose food cans and can be formulated to meet specific requirements, such as resistance to certain solvents or chemicals.
- Polyolefin Coatings: Polyolefin coatings, such as polyethylene and polypropylene, are used for their excellent inertness and resistance to a wide range of chemicals. They are often employed in cans designed for specific food products, like those with high salt content.
- Vinyl Coatings: Vinyl coatings provide good chemical resistance and flexibility. They are suitable for various food products and are often chosen for their good performance at lower temperatures.
Importance of Coating Integrity in Preventing Food Contamination
The integrity of the can coating is paramount in ensuring food safety. Any breach in the coating, such as scratches, pinholes, or delamination, can expose the can material to the food product. This exposure can lead to several adverse outcomes.
- Corrosion: Exposed metal surfaces are susceptible to corrosion, which can generate rust and other corrosion products that contaminate the food.
- Metal Migration: The can material (typically steel or aluminum) can leach into the food, introducing undesirable metal ions. The amount of metal migration depends on several factors, including the type of food, the acidity of the food, the temperature of storage, and the duration of storage.
- Food Spoilage: In some cases, a compromised coating can lead to food spoilage due to the interaction between the food and the can material.
A comprehensive quality control program, including visual inspections and tests like the “button test” (where a small section of the coating is removed to assess its adhesion), is essential to ensure the integrity of the coating.
Role of Coatings in Protecting the Can from Corrosion
Coatings are fundamental in protecting the can from corrosion. Corrosion is an electrochemical process that occurs when the can material reacts with the food product, leading to degradation of the metal. Coatings act as a barrier, preventing direct contact between the food and the can material.
- Barrier Protection: The primary function of the coating is to create a physical barrier, isolating the can material from the corrosive components of the food. This is particularly crucial for acidic foods, which accelerate corrosion.
- Chemical Resistance: Coatings are formulated to resist degradation by the specific chemicals present in the food product. Different coatings are selected based on their resistance to acids, alkalis, and other substances.
- Adhesion: Good adhesion of the coating to the can material is essential. If the coating does not adhere properly, it can peel or flake, compromising its protective function.
- Flexibility: Coatings must be flexible enough to withstand the stresses of can manufacturing and handling without cracking or delaminating.
For example, in a tomato-based product, which is highly acidic, a robust epoxy coating is used. This coating forms a barrier to prevent the acid from attacking the steel can, thus preventing rust and maintaining the integrity of the product. Without this coating, the can would corrode rapidly, rendering the product unsafe for consumption.
Can Dimensions and Standardization
Canned food products are available in a vast array of sizes, each designed to meet specific consumer needs and processing requirements. Standardization of can dimensions is crucial for efficient manufacturing, filling, labeling, and distribution. This section will explore the standard can sizes, the systems used to identify them, and how these standards vary across different regions.
Standard Can Sizes and Their Uses
The food industry relies on a set of established can sizes, each suited to particular food products and serving different market segments. These standardized dimensions ensure compatibility with filling machinery, labeling equipment, and retail shelving. The table below provides a comprehensive overview of common can sizes, along with their typical applications.
Diameter (inches) | Height (inches) | Nominal Volume (fluid ounces) | Common Uses |
---|---|---|---|
2 1/16 | 2 1/16 | 5.0 | Single-serving fruits, vegetables, and soups. |
2 11/16 | 4 | 15.0 | Fruits, vegetables, and condensed soups for family-sized portions. |
3 1/16 | 4 1/16 | 28.0 | Larger-sized fruits, vegetables, and beans. |
4 1/4 | 7 | 100.0 | Institutional and foodservice applications; larger volumes of vegetables, fruits, and sauces. |
Can Size Labeling and Identification
A standardized system exists for identifying can sizes, typically using a three-digit number to represent the diameter and height. This system, often referred to as the “inch-based” system, uses the following format:
DDD x EEE
Where:
- DDD represents the diameter in inches and sixteenths of an inch.
- EEE represents the height in inches and sixteenths of an inch.
For instance, a can labeled “307 x 409” would have a diameter of 3 and 7/16 inches and a height of 4 and 9/16 inches. This labeling system provides a clear and concise way to communicate the dimensions of a can, simplifying communication throughout the supply chain.
Regional Standards Comparison
While the inch-based system is prevalent in North America, other regions may utilize slightly different standards or metric measurements. Differences are minor, focusing on details like the tolerances allowed in dimensions.
- North America: The inch-based system, as described above, is the primary standard.
- Europe: Metric measurements may be used in addition to or instead of the inch-based system. Variations exist based on national standards bodies.
- Asia: Both inch-based and metric systems are utilized, with specific standards varying by country and influenced by the products being packaged and international trade.
Understanding these regional variations is essential for global food manufacturers and distributors to ensure compatibility with local regulations and consumer expectations.
Food Preservation and Sterilization
Canning relies heavily on preservation techniques to ensure food safety and extend shelf life. The core principle involves eliminating or inactivating microorganisms and enzymes that cause spoilage. This is achieved through a combination of heat treatment, airtight sealing, and, in some cases, the addition of preservatives. The primary goal is to create a shelf-stable product that can be stored at room temperature for extended periods without deterioration.
Principles of Food Preservation in Canned Foods
Food preservation in canned foods hinges on several key principles. These principles work in concert to prevent spoilage and maintain the quality of the food.
- Microbial Inactivation: The most critical aspect is the elimination of spoilage microorganisms, including bacteria, yeasts, and molds. This is primarily achieved through heat sterilization, which denatures microbial proteins and disrupts cellular functions, leading to their inactivation. The specific heat treatment depends on the type of food and the likely presence of heat-resistant microorganisms like
-Clostridium botulinum*. - Enzyme Inactivation: Enzymes naturally present in food can continue to catalyze reactions that degrade the food’s quality, even after the food has been processed. Blanching (briefly heating the food before canning) and sterilization inactivate these enzymes, preventing undesirable changes in flavor, texture, and color.
- Airtight Sealing: The hermetic seal of the can is crucial. It prevents the re-entry of microorganisms and oxygen, which are essential for microbial growth and oxidative reactions that lead to spoilage. The can’s seal must remain intact throughout storage and handling.
- Control of Water Activity: While not directly manipulated in most canning processes, the water activity (aw) of the food is indirectly affected. Sterilization reduces the aw by removing water, which can also help inhibit microbial growth. Foods with lower aw values are generally more shelf-stable.
- pH Adjustment: The pH of the food can influence the type of microorganisms that can grow. Acidic foods (pH < 4.6), such as fruits, are generally easier to sterilize because fewer microorganisms can survive. In contrast, low-acid foods (pH > 4.6), such as vegetables and meats, require more rigorous heat treatments to eliminate
-Clostridium botulinum*.
Sterilization Process for Food Safety
The sterilization process is a carefully controlled procedure designed to eliminate harmful microorganisms and ensure the safety of canned foods. It is a critical step in achieving a commercially sterile product.
- Pre-processing: This step involves preparing the food for canning. This can include washing, peeling, cutting, blanching, and adding ingredients. Blanching, as mentioned before, is crucial for enzyme inactivation.
- Filling and Exhausting: The prepared food is filled into the cans, leaving appropriate headspace (empty space at the top of the can). Exhausting removes air from the headspace to reduce the pressure inside the can during sterilization and to minimize the risk of corrosion. Exhausting methods include steam exhausting and hot filling.
- Sealing: The cans are hermetically sealed, creating an airtight barrier that prevents contamination. This step is crucial for maintaining sterility.
- Sterilization (Heat Treatment): The sealed cans are subjected to a specific heat treatment in a retort (pressure cooker). The temperature and duration of the sterilization process are determined by the food type, the can size, and the target microorganisms (particularly
-Clostridium botulinum*). - Cooling: After sterilization, the cans are cooled rapidly to prevent overcooking and to minimize the growth of any surviving microorganisms. Cooling is typically done with water.
- Inspection and Storage: The cans are inspected for defects, such as leaks or swelling. Properly sterilized cans are then stored in a controlled environment.
Role of Heat Treatment in Extending Shelf Life
Heat treatment is the cornerstone of extending the shelf life of canned products. It achieves this primarily through microbial inactivation and enzyme deactivation.
- Microbial Destruction: Heat sterilization destroys spoilage microorganisms and pathogens, preventing their growth and multiplication. The severity of the heat treatment is tailored to the food’s characteristics and the most heat-resistant microorganisms present.
- Enzyme Inactivation: Heat treatment inactivates enzymes that can degrade the food’s quality over time, such as those causing browning or softening.
- Shelf-Stable Products: The combination of microbial inactivation, enzyme deactivation, and airtight sealing creates a shelf-stable product that can be stored at room temperature for extended periods, often for years.
- Examples: Consider canned tomatoes. The heat treatment destroys the microorganisms that cause spoilage, such as molds and yeasts. Without heat treatment, these tomatoes would spoil within days. Similarly, canned meats benefit from heat treatment, destroying pathogens like
-Clostridium botulinum*, ensuring safety and preventing botulism.
Factors Affecting the Sterilization Process
Several factors influence the effectiveness of the sterilization process. Careful control of these factors is essential to ensure food safety and product quality.
Do not overlook the opportunity to discover more about the subject of food trucks baton rouge.
- Food Characteristics: The type of food significantly impacts the sterilization process.
- pH: Acidic foods (pH < 4.6) require less severe heat treatment than low-acid foods (pH > 4.6).
- Composition: The food’s composition (e.g., fat content, sugar content) affects heat penetration and microbial resistance.
- Viscosity: The viscosity of the food influences heat transfer. Thicker foods require longer sterilization times.
- Initial Microbial Load: The number of microorganisms present in the food before sterilization influences the required heat treatment. Foods with higher initial loads require more intensive sterilization.
- Can Size and Shape: The size and shape of the can affect the rate of heat penetration. Larger cans and cans with irregular shapes take longer to heat.
- Retort Operation: The retort’s operating parameters, such as temperature, pressure, and steam distribution, must be carefully controlled. Inadequate retort operation can lead to under-processing.
- Heat Penetration: The rate at which heat penetrates the food inside the can is crucial. Factors influencing heat penetration include the food’s composition, the can’s size and shape, and the agitation during sterilization.
- Target Microorganism: The most heat-resistant microorganism of concern determines the required sterilization process. For low-acid foods,
Clostridium botulinum* is the critical organism.
- Process Validation: The sterilization process must be validated to ensure it effectively eliminates target microorganisms. This often involves temperature distribution studies and microbial challenge tests.
- Temperature and Time: The specific combination of temperature and time (F0 value) is crucial for effective sterilization. The F0 value represents the equivalent time at 250°F (121.1°C) required to achieve a specific level of microbial inactivation.
F0 = Time at Temperature / D-value (of target organism at that temperature)
Where D-value is the decimal reduction time (the time required to reduce the microbial population by 90% at a specific temperature).
Can Defects and Quality Control
The integrity of food cans is paramount for ensuring food safety and maintaining product shelf life. Rigorous quality control measures are essential throughout the manufacturing process to identify and mitigate potential defects. These defects, if left unaddressed, can compromise the hermetic seal, leading to spoilage, contamination, and potential health risks.
Common Can Defects
Several types of defects can occur during the manufacturing or handling of food cans. These defects can compromise the can’s ability to protect its contents.
- Dents: These are indentations on the can body, which can result from impacts during handling, transportation, or filling. Severe dents can compromise the can’s structural integrity and potentially damage the protective coatings.
- Corrosion: This is the degradation of the can material, usually metal, due to chemical reactions with the environment or the food product. Corrosion can lead to leaks and contamination. This can be visible as rust on the exterior or pitting on the interior.
- Seam Imperfections: These are defects in the seams that join the can body, bottom, and lid. Common seam imperfections include:
- False Seams: These are seams that are not properly interlocked, leaving gaps that allow leakage and contamination.
- Cutovers: These occur when the can body material is cut or torn during the seaming process, compromising the seal.
- Sharp Seams: These are seams with sharp edges that can weaken the can’s structure and potentially lead to failure.
- Coating Defects: Imperfections in the protective coatings applied to the interior and exterior of the can can expose the metal to corrosion. Examples include pinholes, scratches, and uneven coating thickness.
- Lid Defects: Defects in the lid, such as improper sealing or damage, can compromise the hermetic seal and allow for contamination.
Methods Used for Quality Control in Food Can Manufacturing
Quality control is implemented throughout the can manufacturing process to ensure that defects are minimized and that the final product meets stringent quality standards. This includes raw material inspection, in-process checks, and final product inspection.
- Incoming Material Inspection: Raw materials, such as steel sheets and coatings, are inspected for quality and conformity to specifications before they are used in the manufacturing process. This includes checking for defects like rust, surface imperfections, and coating integrity.
- In-Process Inspection: Inspections are conducted at various stages of the manufacturing process to identify and correct defects as they arise. These include:
- Body Formation Inspection: Checking for proper dimensions, shape, and integrity of the can body.
- Seaming Inspection: Monitoring the seaming process to ensure proper seam formation and seal integrity.
- Coating Inspection: Verifying the quality and uniformity of the interior and exterior coatings.
- Final Product Inspection: Finished cans are subjected to a series of tests to ensure they meet all quality requirements. These include:
- Visual Inspection: Examining the cans for dents, corrosion, and other visible defects.
- Seam Inspection: Assessing the quality of the seams using various methods (see below).
- Leak Testing: Testing the cans for leaks using methods such as vacuum testing or pressure testing.
- Dimensional Checks: Measuring the can dimensions to ensure they meet specifications.
- Performance Testing: Evaluating the can’s performance under various conditions, such as drop tests and stacking tests.
- Statistical Process Control (SPC): SPC is a statistical method used to monitor and control the manufacturing process. Data is collected and analyzed to identify trends and variations in the process, allowing for timely adjustments to prevent defects.
Impact of Can Defects on Food Safety and Shelf Life
Can defects can have serious consequences for food safety and shelf life. A compromised can may result in the product being unsafe for consumption.
- Food Spoilage: Defects, particularly those that compromise the hermetic seal, allow microorganisms to enter the can and multiply, leading to food spoilage. This can result in changes in the product’s appearance, taste, and odor.
- Microbial Contamination: Leaks can allow bacteria, yeasts, and molds to enter the can, potentially causing foodborne illnesses. Pathogenic bacteria, such as
-Clostridium botulinum*, can produce toxins in the anaerobic environment of a sealed can, leading to botulism. - Reduced Shelf Life: Even minor defects can reduce the shelf life of the product. The presence of oxygen or moisture can accelerate spoilage and reduce the product’s quality over time.
- Health Risks: Consumption of food from defective cans can pose serious health risks. Bacterial contamination can cause food poisoning, while chemical reactions between the food and the can material can lead to the formation of harmful substances.
Process for Inspecting Can Seams Using Visual and Non-Destructive Testing Methods
Seam inspection is a critical component of quality control in can manufacturing. Various methods are used to assess the integrity of can seams.
- Visual Inspection: This is a basic but essential step. Trained inspectors visually examine the seams for any obvious defects.
- Appearance: Checking for wrinkles, sharp edges, and any signs of damage.
- Dimensions: Measuring seam dimensions, such as seam width, overlap, and countersink, to ensure they meet specifications.
- Equipment: Use of a seam micrometer and a seam projector, that projects an enlarged image of the seam profile.
- Non-Destructive Testing Methods: These methods do not damage the can and can be used to assess seam integrity.
- Seam Tear-Down: This involves carefully dismantling the seam to measure the various seam components, such as the body hook, cover hook, and overlap.
- Cross-Sectioning: Cutting a cross-section of the seam to examine its structure under a microscope. This allows for detailed analysis of the seam profile and the presence of any defects.
- Ultrasonic Testing: This method uses ultrasonic waves to detect defects in the seam. The waves are transmitted through the seam, and any discontinuities or imperfections will cause a change in the reflected signal.
- Example: A large food processing company implemented a comprehensive seam inspection program, including automated vision systems and regular tear-down analyses. This resulted in a significant reduction in seam defects and a decrease in product recalls due to can failures.
Environmental Considerations and Sustainability
The food can industry, while essential for food preservation and distribution, presents several environmental challenges. From the extraction of raw materials to manufacturing processes and end-of-life disposal, each stage impacts the environment. Addressing these impacts and promoting sustainable practices is crucial for minimizing the industry’s ecological footprint and ensuring long-term viability.
Environmental Impact of Food Can Manufacturing
The manufacturing of food cans has a multifaceted environmental impact. This includes resource depletion, energy consumption, greenhouse gas emissions, and waste generation. The extraction of raw materials, such as tin, steel, and aluminum, often involves mining activities that can lead to habitat destruction, soil erosion, and water pollution. Manufacturing processes, including smelting, rolling, and coating, require significant energy, primarily from fossil fuels, contributing to greenhouse gas emissions.
The disposal of manufacturing byproducts and the potential for chemical releases during production further exacerbate the environmental burden.
Recyclability of Food Cans and the Recycling Process
Food cans are highly recyclable, particularly those made from steel and aluminum. Recycling these materials reduces the demand for virgin resources, conserves energy, and lowers greenhouse gas emissions compared to manufacturing new cans from scratch. The recycling process involves several key steps: collection, sorting, processing, and manufacturing.The process begins with the collection of used food cans through various channels, including curbside recycling programs, drop-off centers, and deposit-refund systems.
Collected cans are then transported to material recovery facilities (MRFs) where they are sorted from other recyclables. This sorting process often utilizes magnets to separate steel cans from other materials, such as aluminum and plastics. Aluminum cans are sorted using eddy current separators.Once sorted, the cans are processed. Steel cans are typically shredded and then melted in electric arc furnaces to produce new steel.
Aluminum cans are similarly shredded and melted, often in dedicated aluminum recycling facilities. The molten metal is then cast into ingots or sheets, which can be used to manufacture new cans or other products.The recycling of steel cans saves approximately 74% of the energy and reduces air pollution by 86% compared to producing steel from virgin iron ore. Recycling aluminum cans saves about 95% of the energy needed to make new aluminum from bauxite ore and significantly reduces greenhouse gas emissions.
Sustainable Practices in Food Can Production
The food can industry is increasingly adopting sustainable practices to minimize its environmental impact. These practices encompass various aspects of the production process, from material sourcing to waste management.Examples of sustainable practices include:
- Using Recycled Materials: Incorporating recycled steel and aluminum into can manufacturing reduces the demand for virgin materials and lowers energy consumption. For instance, many can manufacturers utilize steel with a high recycled content.
- Energy Efficiency: Implementing energy-efficient technologies and processes, such as using electric arc furnaces and optimizing production lines, can significantly reduce energy consumption and greenhouse gas emissions.
- Reducing Water Usage: Employing water-saving techniques, such as closed-loop cooling systems and efficient cleaning methods, can minimize water consumption.
- Waste Reduction and Recycling: Implementing comprehensive waste management programs, including recycling and reducing waste generation, helps to minimize landfill waste and recover valuable materials.
- Sustainable Coatings: Utilizing environmentally friendly coatings and liners, such as those that are free of bisphenol A (BPA) and use water-based formulations, reduces the risk of chemical contamination and environmental impact.
- Optimized Transportation: Streamlining logistics and optimizing transportation routes reduces fuel consumption and greenhouse gas emissions associated with the distribution of cans.
- Lightweighting: Reducing the weight of cans through design and material optimization lowers the amount of material used and the energy required for manufacturing and transportation.
Ways to Reduce the Environmental Footprint of Food Can Manufacturing, Food can structures
Several strategies can be employed to further reduce the environmental footprint of food can manufacturing.
- Increase the use of recycled materials: Maximize the proportion of recycled steel and aluminum used in can production.
- Invest in renewable energy sources: Transition to renewable energy sources, such as solar and wind power, to reduce reliance on fossil fuels.
- Implement closed-loop manufacturing processes: Establish closed-loop systems to recycle water and other resources within the manufacturing process.
- Develop eco-friendly coatings and liners: Continue research and development of sustainable coatings and liners that minimize environmental impact.
- Optimize can design for lightweighting: Design cans to be as light as possible while maintaining structural integrity.
- Improve waste management and recycling programs: Implement robust waste management programs and promote the recycling of all manufacturing byproducts.
- Collaborate with suppliers and customers: Work with suppliers and customers to promote sustainable practices throughout the entire supply chain.
- Adopt life cycle assessments: Conduct life cycle assessments to identify environmental hotspots and areas for improvement.
Future Trends and Innovations
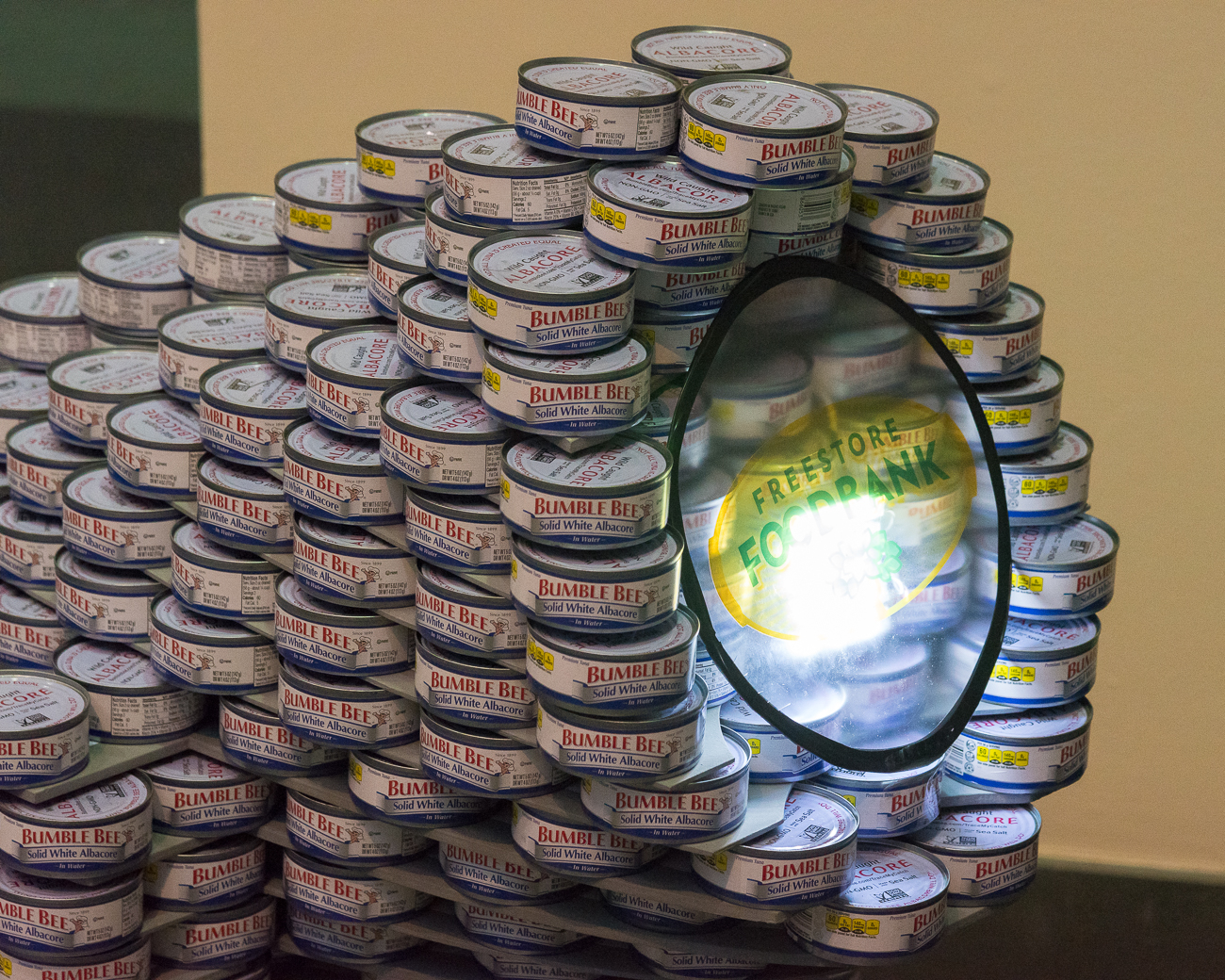
The food can industry is continuously evolving, driven by consumer demands for convenience, sustainability, and enhanced food safety. Innovations in materials, manufacturing processes, and smart technologies are reshaping the landscape of food packaging. This section explores the emerging trends and future directions of food can design and manufacturing.
Emerging Trends in Food Can Design and Manufacturing
Several key trends are shaping the future of food can design and manufacturing. These trends reflect the industry’s response to evolving consumer expectations and environmental concerns.
- Lightweighting: Reducing the weight of cans to minimize material usage and transportation costs. This is achieved through the use of thinner materials and innovative design. For example, some manufacturers are using high-strength steel alloys to reduce can wall thickness while maintaining structural integrity.
- Shape Optimization: Exploring new can shapes and designs to improve shelf appeal, optimize space utilization, and enhance consumer convenience. This includes ergonomic designs and easy-open features.
- Digital Printing: Implementing digital printing technologies for high-quality graphics, personalized packaging, and on-demand printing, enabling greater flexibility and customization.
- Automation and Robotics: Increasing the use of automation and robotics in manufacturing processes to improve efficiency, reduce labor costs, and enhance precision. This includes automated can assembly, filling, and sealing systems.
- Sustainable Manufacturing Practices: Adopting environmentally friendly manufacturing processes, such as reducing energy consumption, minimizing waste, and using recycled materials.
Innovations in Can Materials and Coatings
The development of new materials and coatings is crucial for improving the performance, sustainability, and functionality of food cans. Several innovations are currently being explored.
- Alternative Materials: Exploring alternative materials to replace traditional tinplate steel, such as aluminum, bio-based plastics, and composite materials. Aluminum offers lightweight properties and recyclability, while bio-based plastics can reduce the reliance on fossil fuels.
- Advanced Coatings: Developing advanced coatings with enhanced barrier properties, improved corrosion resistance, and greater flexibility. These coatings can extend shelf life, protect food quality, and enable new design possibilities. Examples include coatings with improved scratch resistance and those designed for microwave-safe applications.
- Recycled Content: Increasing the use of recycled materials in can manufacturing to reduce environmental impact and promote circular economy principles.
- Smart Coatings: Integrating smart coatings with sensors that can detect spoilage, indicate freshness, and provide information on food safety.
The Role of Smart Packaging and Its Application to Food Cans
Smart packaging technologies are revolutionizing the food industry by providing real-time information about product quality, safety, and traceability. Food cans are increasingly incorporating these technologies.
- Active Packaging: Incorporating active packaging components that interact with the food to extend shelf life, improve food safety, and enhance sensory properties. This can include oxygen scavengers, moisture absorbers, and antimicrobial agents.
- Intelligent Packaging: Integrating intelligent packaging features, such as sensors and indicators, that provide information on product condition, temperature, and tampering.
- Near Field Communication (NFC) and QR Codes: Utilizing NFC tags and QR codes to provide consumers with access to product information, including origin, ingredients, nutritional information, and recycling instructions.
- Traceability: Implementing track-and-trace systems to enable complete traceability of food products throughout the supply chain, enhancing food safety and reducing the risk of recalls.
Future Food Can Design: Sustainability and Consumer Convenience
The future of food can design will prioritize sustainability and consumer convenience. A hypothetical future food can design could incorporate the following features:
- Material: A can made from 100% recycled aluminum, with a thin, high-performance coating derived from bio-based materials, ensuring recyclability and reducing reliance on virgin materials.
- Shape and Design: A re-sealable, easy-open design with a built-in spoon or fork, promoting portion control and convenience. The can would have an ergonomic shape for easy handling and storage.
- Smart Technology: An integrated smart label with an NFC tag and QR code, providing access to product information, recipes, and recycling instructions. A built-in sensor would monitor the food’s freshness and indicate spoilage.
- Manufacturing: The manufacturing process would be highly automated, using renewable energy sources and minimizing waste. The production would be localized to reduce transportation emissions.
- Sustainability Features: The can would be designed for easy disassembly and recycling, with clear instructions for consumers. The packaging would be part of a closed-loop recycling system, ensuring that the materials are reused.
This future food can design exemplifies a shift towards a circular economy, prioritizing resource efficiency, environmental protection, and consumer-centric features. This vision reflects the evolving demands of consumers and the growing need for sustainable packaging solutions.
Final Summary
In conclusion, the journey through food can structures reveals a fascinating blend of engineering, materials science, and food preservation techniques. From the selection of materials to the intricacies of the manufacturing process, every aspect is carefully considered to ensure the safety and longevity of the contents. As the industry continues to evolve, driven by sustainability and consumer demands, food can structures will undoubtedly continue to play a vital role in the global food supply chain.
The innovations in design, materials, and manufacturing processes will undoubtedly lead to even more efficient, sustainable, and consumer-friendly packaging solutions in the years to come.